This article describes one of the most valuable tools in the EMC engineer’s “bag of tricks” – the high-frequency current probe. Current probes are invaluable for measuring high-frequency common-mode (or “antenna”) currents flowing on wires or cables. Experience has proven that poorly terminated (bonded or filtered) cables are the no. 1 cause for radiated emissions failures at a test facility. By measuring the common-mode (CM) currents (sometimes referred to as “antenna” currents) on these cables it’s possible to troubleshoot and apply fixes to a product right there in your development lab. You can also predict, to a good degree of accuracy, whether a given cable current will pass or fail in the measurement chamber. This will save you tons of time trying to apply fixes at the test facility while the clock is ticking away your test time. I’ll also show you several ways to create do-it-yourself (DIY) probes that are quick to make and very useful in a pinch.
COMMON-MODE CURRENTS
Let’s consider CM currents and how they are generated, because it is not intuitive as to how current may travel in the same direction through both the signal and signal-return wires in a cable or PC board. Referring to Figure 1, note that due to finite impedance in any grounding system, including circuit board signal/power return planes, there will be a voltage difference between any two points within that return plane. This is denoted by VGND1 and VGND2 in the figure. This difference in potential will drive CM currents through common cabling or circuit traces between circuits or sub-systems. In addition, unbalanced geometries—for example, different lengths or path routings for high-speed differential pairs—can create voltage sources that drive associated CM currents. Finally, routing a high-speed clock trace across a split in the return plane or referencing it to multiple planes, can also be a source of CM current. Because the current phasors in Figure 1 are additive, the resulting radiated phasor may be quite large compared to those generated by differential-mode (DM), or signal currents, which are opposite in direction, and so tend to cancel. Therefore, CM emissions tend to be more of an issue than DM emissions.
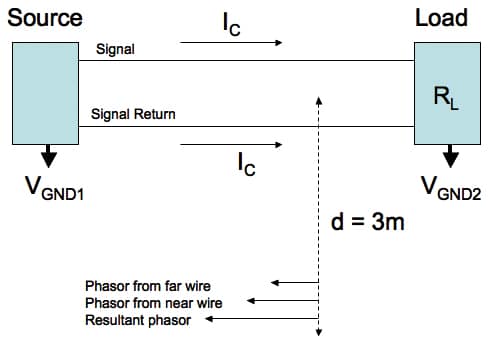
CURRENT PROBES: THEORY OF OPERATION
The RF current probe is an “inserted-primary” type of radio frequency current transformer. When the probe is clamped over the conductor or cable in which current is to be measured, the conductor forms the primary winding. The clamp-on feature of this probe enables easy placement around any conductor or cable. This is essentially a broadband high-frequency transformer. High-frequency currents can be measured in cables without physically disturbing the circuit.
Since the current probe is intended for “clamp-on” operation, the primary shown in Figure 2 is actually the electrical conductor in which CM currents are to be measured. This primary is considered as one turn since it is assumed that the CM currents flow through the conductor and return to the source via a return conductor such as a frame, common ground plane, or earth. On some current probe models the secondary output terminals are resistively loaded internally to provide substantially constant transfer impedance over a wider frequency range.

COMMERCIAL CURRENT PROBES
While commercial current probes are pricey, the advantage is that they can open up and snap around a cable, rather than having to be threaded onto the cable to be measured. See Figure 3. They are also a lot more rugged and can take a lot of abuse as compared to the “do-it-yourself” (DIY) versions below. Finally, they are also accurately characterized, allowing very precise measurements of cable currents.
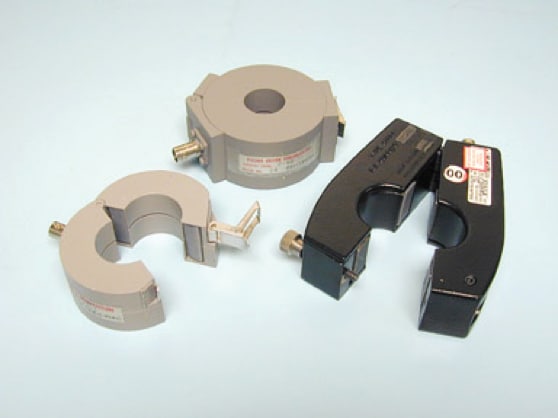
DIY CURRENT PROBES
In a pinch, you can make your own current probe. Examples of several DIY probes are shown in Figures 4 and 5. I typically try to find a ferrite toroid or clamp-on core that offers good high-frequency characteristics in the 10 to 1000 MHz range. Winding a few (not too critical) turns and terminating with a coax connector is all you need. Keeping the turns as far apart as possible (as in Figure 4) will reduce inter-winding capacitance and yield better results at the higher frequencies. This is one of the largest drawbacks in performance of the clamp-on ferrites (as in Figure 5).
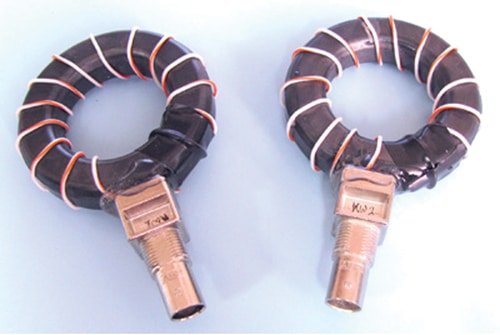
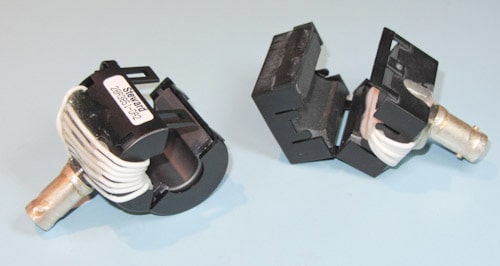
TRANSFER IMPEDANCE
The CM current (Ic) in microamps in the conductor under test is determined from the reading of the current probe output (V) in microvolts divided by the current probe transfer impedance (ZT).
I = V/ZT (1)
or, in dB
I(dBuA) = V(dBuV) – ZT(dBΩ) (2)
The typical transfer impedance of the current probe throughout the frequency range is determined by passing a known RF current (ic) through the primary test conductor and noting the voltage (V) developed across a 50-Ohm load. Then,
ZT = V/Ic (in standard units) (3)
or
ZT(dBΩ) = V(dB∫V) – Ic(dB∫A) (4)
The Fischer F-33-1 probe is a commonly used troubleshooting tool and has a flat frequency response from 2 to 250 MHz (Figure 6). The transfer impedance is about 5Ω (approximately +14 dBΩ on the graph), therefore, a 1 uA current will produce a 5 uV output voltage from the current probe.

PROBE CALIBRATION
The accurate calibration of RF current probes is a complex process. Characterization is a more correct term to use than calibration. The probe must be properly characterized to reflect how the user uses the probe. Probe manufacturers usually sell a calibration fixture that attempts to maintain a 50Ω impedance. A 50Ω load is connected to the output port and a calibrated RF generator (or network analyzer) is connected to the input port. The probe to be characterized is clamped around the fixture and the frequency is swept while measuring the probe output.
My test setup was a little more rudimentary (Figure 7), but for troubleshooting purposes, it’s good enough. I used a short piece of stiff wire across the output port with a 50Ω resistive load in series. I then adjusted the generator for zero dBm – a convenient amount. This is equivalent to an output voltage of 224 mV (or 73 dBuA of current) into 50Ω. The actual generator output doesn’t matter, so long as the resulting probe voltage is large enough to be seen readily in the receiver or spectrum analyzer. I monitored the probe output with a Thurlby Thander TTi PSA2701T handheld spectrum analyzer.
Knowing the current through the wire in dBuA and the probe output in dBuV, the transfer impedance may be plotted graphically by subtracting: V(dBuV) – ic(dBuA) (expressed in dB). In this case, zt(dBΩ) = V(dBuV) – 73. While this may be useful for educational purposes, I wouldn’t be too inclined to use the DIY probes to predict “pass/fail”, as described further down. However, because they compare favorably to the commercial probes as far as output voltage, I believe (and have proven in practice) that they are completely suited for troubleshooting. You only need to know whether an EMC design fix made the cable current better or worse.

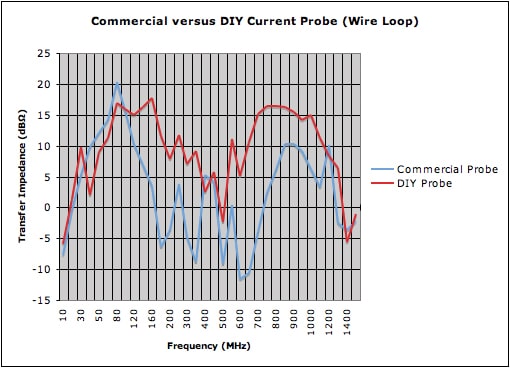
PREDICTING PASS/FAIL
It is possible to predict whether a particular cable will pass or fail radiated emissions by measuring the CM current at the offending frequency, reading off the transfer impedance of the probe, Zt (dBΩ) in Figure 6, and solving for ic (using Equation 2 above). Plugging ic(Amps) into Equation 5 will calculate the E-field level in V/m. The length of the cable is L(m) and the offending harmonic frequency is f(Hz). Use a test distance, d, of either 3 or 10m to predict the outcome at those test distances.
Once you’ve determined a particular cable has CM currents that may cause a RE failure, you should to examine the connector where the cable is attached to the product enclosure. Very often, I find poor or non-existent bonding between the connector shield and enclosure shield. These points must be bonded well to permit the CM currents to flow back to their source within the product, avoiding associated cable radiation. Please refer to my previous articles on troubleshooting radiated emissions for more information (references below).
REAL-WORLD TROUBLESHOOTING EXAMPLE
As previously mentioned, one of the most common sources of radiated emissions is due to poorly bonded connectors mounted on shielded product enclosures. This occurs especially if the connectors are circuit board mounted and penetrate loosely through the shielded enclosure. Poorly bonded connectors allow internally generated CM currents to leak out and flow on the outside of I/O, mouse or keyboard cables. This will also allow ESD discharges inside the product – more bad news. If these currents are allowed out of the enclosure, the attached cables will act as radiating antennas – often resonating around 300 MHz, due to their typical 1m length.
This was the case for a new digitizing oscilloscope prototype I worked on recently. The I/O connectors were all soldered onto the PC board and the board was fastened to the rear half of the enclosure. The connectors simply poked up through cutouts in the rear metal shield.
While using a current probe to measure the CM current flowing on the outside of the USB cable under test, I simply jammed the screwdriver blade of my Swiss Army knife between the connector bonding fingers and metal chassis enclosure and was able to drop the overall cable currents by 10 to 15 dB.
The solution was to fabricate a custom shim with spring-fingers that would slip over all the connectors creating a firm bond between the connector ground shell and inside of the shielded enclosure. More and more low-cost products are relying on PC board mounted I/O connectors as a cost-cutting measure. Any time you see this, be prepared to carefully examine the bonding between the connector ground shell and the shielded enclosure.
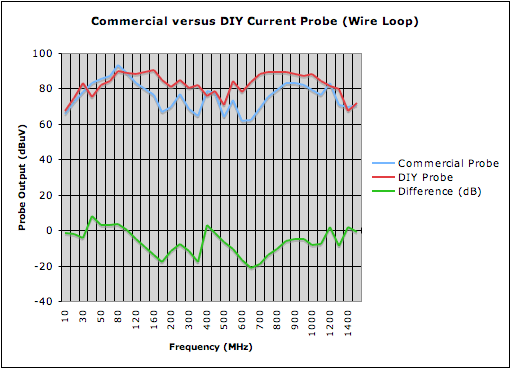

TROUBLESHOOTING TIPS USING CURRENT PROBES
Here are a few troubleshooting tips using current probes.
1. When evaluating the harmonics on a cable by using a current probe, if sliding the probe back and forth changes the harmonic levels, part of the coupling may be near-field, rather than conducted.
2. When using a pair of current probes; one on each of two cables, if the harmonics are the same in each, the source is in the middle. If one cable has stronger harmonics, then you’ll want to work on that side first. See Figure 12 below.
3. Measuring the currents on two suspect legs of a dipole should read the same. Placing the two suspect legs through the same current probe should cause a big decrease due to current cancellation. See Figure 12 below.
4. When measuring video cable currents and large cable movements cause big changes in amplitude, the coupling is likely inductive – otherwise, it’s more likely conductive.
5. If you suspect inductive coupling, the phase at the victim will be 180-degrees from the source. This may be observed on an oscilloscope with H-field probes or current probes. Try syncing the scope trigger at the source using a scope probe.
My colleague, Doug Smith, has many more examples on how to use current probes for measuring cable and PC board resonances, injecting pulses for troubleshooting, interpreting the relative phase of common-mode currents and troubleshooting ESD issues. Refer to the references below.
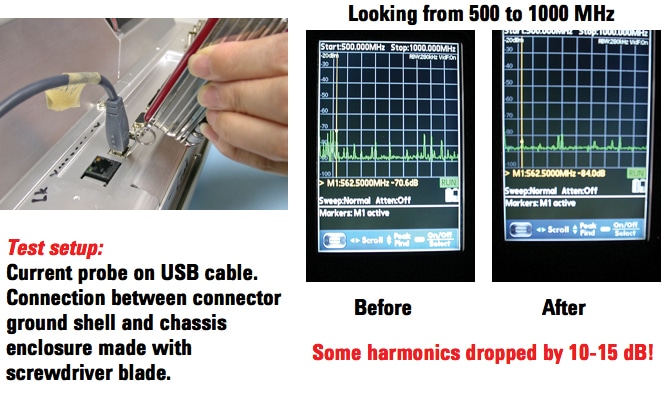

SUMMARY
Use of a current probe is vital during the troubleshooting process. Poorly bonded cable connectors can be readily identified and fixed. The radiated E-field from a product I/O cable may be calculated by measuring the high-frequency common-mode currents flowing in the cable. All this may be performed right at the designer’s workbench and without the expense of a third-party test facility or shielded chamber.
REFERENCES – PAPERS
[1] Mat Aschenberg & Charles Grasso, Radiation from Common-Mode Currents – Beyond 1 GHz (Three Methods Compared)
[2] Dave Eckhardt, Homebrew Clamp-On Current Probe, private correspondence (January 2009), Email: [email protected].
[3] Jasper Goodblood, Electromagnetic Compatibility, 1990, Prentice Hall, pages 31-34.
[4] Michel Mardiguian, EMI Troubleshooting Techniques, McGraw-Hill, 2000, pages 39-49.
[5] Montrose & Nakauchi, Testing for EMC Compliance, 2004, Wiley Interscience, pages 116-124, 143-145, and 159-161.
[6] Henry Ott, Electromagnetic Compatibility Engineering, Wiley, 2009, pages 690-693.
[7] Henry Ott, Measuring Common-Mode Currents on Cables, www.hottconsultants.com/techtips/tips-cm.html
[8] Clayton Paul, Introduction to Electromagnetic Compatibility (2nd Edition), Wiley Interscience, 2006, pages 518-532.
[9] Ridao, Carrasco, Galvin and Franquelo, Implementation of low cost current probes for conducted EMI interference measure in Power Systems, EPE 1999 (Lausanne).
[10] H. Ward Silver, Hands-On Radio column, Detecting RF – Part 2, QST, August 2011, page 54-55.
[11] Doug Smith, Current Probes, More Useful Than You Think, IEEE EMC Symposium 1998, http://emcesd.com/pdf/iprobe98.pdf.
[12] Doug Smith, The Two Current Probe Puzzle, http://emcesd.com/tt061999.htm.
[13] Doug Smith, Using Current Probes to Inject Pulses for Troubleshooting, http://emcesd.com/tt2007/tt120307.htm Part 1, http://emcesd.com/tt2009/tt030309.htm Part 2.
[14] Doug Smith, Using Current Probes to Measure Cable Resonance, http://emcesd.com/tt2008/tt010108.htm.
[15] Doug Smith, Measuring and Interpreting the Relative Phase of Common Mode Currents, http://emcesd.com/tt2008/tt030208.htm.
[16] Doug Smith, Using a Comb Generator with a Pair of Current Probes to Measure Cable Resonance, http://emcesd.com/tt2009/tt110709.htm.
[17] Doug Smith, Using Current Probes to Inject Pulses for Troubleshooting (Board Resonances), http://emcesd.com/tt2010/tt010110.htm.
[18] Doug Smith, Predicting Cable Emissions from Common Mode Current, http://emcesd.com/tt2006/tt030106.htm.
[19] Douglas Smith, High Frequency Measurements and Noise in Electronic Circuits, Van Nostrand Reinhold, 1993, pages 41-44, 159-182, 192-209.
[20] Allen Wolff, Building a Ferrite Core Antenna Current Probe, Technical Correspondence, QST, August 2009, page 53.
[21] Kenneth Wyatt, Troubleshooting Radiated Emissions Using Low-Cost Bench-Top Methods, Interference Technology (ITEM) – EMC Directory & Design Guide 2011, May 2011, page 10-21, https://interferencetechnology.com/uploads/media/Wyatt_DDG11.pdf.
[22] Kenneth Wyatt, Troubleshooting Radiated Emissions – Three Case Studies, InCompliance Magazine, October 2011, http://www.incompliancemag.com/index.php?option=com_content&view=article&id=818:troubleshooting-radiated-emissions-three-case-studies&catid=27:testing&Itemid=136.
REFERENCES – SUPPLIERS
[23] Fischer Custom Communications (FCC), Phone: (310) 303-3300, Email: [email protected], Web: www.fischercc.com. They provide a very wide range of HF current probes – their specialty.
[24] Laird Technologies, Web: http://www.lairdtech.com/Products/EMI-Solutions/. They offer a complete line of ferrite cores and chokes.
[25] Pearson Electronics, Phone: (650) 494-6444, Email: [email protected], Web: www.pearsonelectronics.com. They have a good selection of probes.
[26] Rhode & Schwartz USA, Phone: (888) 837-8772, Email: [email protected], Web: www.rohde-schwartz.us. They have a very limited selection.
[27] Solar Electronics, Phone: (800) 952-5302, Email: [email protected], Web: www.solar-emc.com. They have a limited selection.
[28] Teseq USA, Phone: (732) 417-0501, Email: [email protected], Web: www.teseq.us. They have a very limited selection.
[29] Thurlby Thander Instruments, Phone: +44-1480-412451, Email: [email protected], Web: http://www.tti-test.com/contact-tti.htm. They offer a low-cost handheld spectrum analyzer for under $2,000 USD.
[30] Würth Electronics Midcom, (605) 886-4385, [email protected], www.we-online.com. I used one of their large ferrite cores for my DIY current probe.