Measurement uncertainty for common-mode disturbances can be reduced from about 22 dB to a maximum of a few dB
Mart Coenen, Philips Applied Technologies
Eindhoven, the Netherlands
Introduction
In early 1980, extensive discussions were held within the IEC CISPR/A working group regarding the definition of the impedance characteristics and the RF emission limits attained via the AMN (artificial mains network) measurement technique. As Philips Research was participating in IEC CISPR/A, Philips’ testers carried out measurements at various locations to determine the actual impedances as functions of frequency of mains wall sockets. After collecting this information, an average was derived, which corresponded reasonably well with the impedances: 50 Ω // (50 µH + 5 Ω) listed in the present i.s.o. proposed IEC/CISPR 16 standard.1 Still, it should be noted that these impedance measurements were taken against i.s.o. at the protective earth (PE) terminal of these mains supply wall outlets and were not the impedances impacting the devices/appliances attached to the mains.
At a later date, common-mode impedance measurements were taken from various cable topologies in Japan, Germany, and the Netherlands, which were used to define the Impedance Stabilizing Networks (ISN) for telecom appliances, in IEC CISPR 22, as well as being the fundament for the definition of the Coupling/Decoupling Networks (CDNs) as defined in IEC 61000-4-6, Testing Measurement Techniques–Immunity to conducted disturbances induced by radio-frequency fields. These impedances were also in line with the coupling networks as defined by R. Bersier of the Swiss PTT (Post, Telephone, and Telegraph) administration in the mid-1970s for the RF immunity measurement test setups2 with audio and video appliances.
Using all these domestic, office and industrial common-mode impedance measurements, a mains value of 150 Ω was found—disregarding the cable topologies used or their application(s). Only in those cases where cables are kept at close distance from a conductive surface—e.g., in automotive environments, lower common-mode impedance values down to 50 Ω were measured. Other measurements such as the definition of the electrical fast transient (EFT) burst revealed an asymmetric line(s)-to-PE impedance of 50 Ω in the frequency range from about 1 to 400 MHz.3 To minimize the effect of cable routing on common mode impedance, the use of an absorbing clamp has been introduced in both RF emission and RF immunity test set-ups.4, 5
Application of the Conducted RF Emission Test
In an RF emission test, carried out according to the various IEC CISPR standards, the device or appliance under test is placed on a insulating support 0.4 meter or 0.8 meter in height (or distance) from the metal reference plane (Figure 1). Note that a distinction must be made between equipment normally placed on a floor and table-top equipment. The test of table-top equipment needs to be performed on a wooden table with the setup placed 0.4 meter from a metal wall or from the metal ground reference plane on the floor.
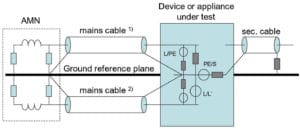
The AMN will be “grounded” to the metal (Faraday cage) wall by a wide metal strip (representing common-mode impedance) 0.4 meter in length running from the wall to the wall socket entry of the AMN. A testing “rule of thumb” holds that 0.4 µH which equals 75j Ω at 30 MHz. Since the device or appliance “source” impedances are typically unknown, for line-to-line, line-to-PE and lines (phase, neutral, and PE) to the ground reference plane (common mode) the likelihood of impedance mismatch on the mains supply cable of the AMN is large. Additionally, the common-mode impedance is influenced by other cables that may be connected to the device or appliance and the way these are terminated in common-mode to the ground reference plane. Here too, the impedance on the secondary lines shall be terminated, in common-mode, by a 150-Ω impedance as defined in References 3 and 6 as ISN and CDN (coupling/decoupling network) (Figure 1).
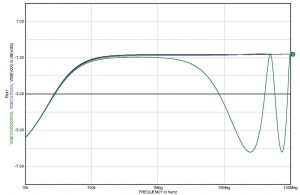
The asymmetric disturbance voltage measurement caused by using an AMN is a combination of the line-to-line, line(s)-to-PE and common-mode currents through the individual AMN impedances, and each of these contributions will be affected separately by the mains cable topology resulting in large variations, i.e., high measurement uncertainties.
When the disturbance source impedances with the device/appliance are unknown, but the transmission line termination is close to or equal to the characteristic impedance of the transmission line, the maximum error is equal to the VSWR and is thus a function of frequency and the physical length of the cable used. The matching between the AMN impedances and the mains cable characteristic impedances is determined by the cross-section of the cable, which should be 50 Ω from line(s)-to-PE and 100 Ω from line-to-line. Such matching is seldom the case with triangular mains cable wire topologies.
According to the common-mode impedance requirements of IEC 61000-4-6, the mains cable shall be kept at 30 mm above the metal ground reference plane to represent (together with the GRP) a characteristic impedance of about 150 Ω at the device/ appliance side. Larger distances from the GRP will yield higher common-mode characteristic impedances, which, in turn, lead to higher mismatch and thus higher uncertainties. In some cases, the mismatch can be infinite. When a characteristic cable impedance of 150 Ω is achieved, but one of the wires of the mains cable is short-circuited while the other two wires (phase/neutral are both terminated with 50 Ω to ground (= 25 Ω phase/neutral to PE), such an infinite mismatch occurs.
When the mains cable is kept 0.4 meter away from the metal plane/ wall/ GRP, the characteristic impedance is about 300 Ω which, together with a common-mode termination of 25 Ω would yield a possible error of 22 dB (12 x).
The mutual coupling between the conductors that occurs in a mains cable and in the wiring following the mains wall outlet is not represented in the AMN. This mutual coupling, particularly between the wires, is close to 50 percent in normal cables but substantially higher in shielded mains cables, particularly where the screen is also used as a PE conductor. Alternatively, consider a situation in which the common-mode termination is 150 Ω at a (modified) AMN and the mains cable is routed 30 mm above a GRP. Then, hardly any mismatch would appear, and the true common-mode disturbance voltage from the DUT could be measured.
Alternative AMN/CDN
When building a modified AMN/CDN that would satisfy; line-to-line, line(s)-to-PE, and the common-mode impedance requirements, there are two plausible options:
- To build the AMN in front of the CDN or
- To build the CDN in front of the AMN.
The CDN M2/36 is essentially a common-mode choke with minimal inductance of 270 µH at 150 kHz (which may decline in inductance versus frequency), and three resistors of 300 Ω in series with AC decoupling capacitors (each linked to the common-mode measurement port and typically terminated by 50 Ω). At the rear of the CDN, a decoupling function shall be provided, which can be represented by a “standard” AMN.
Building this network in the alternative fashion with the AMN in front of the CDN would require large components at the common-mode measurement load side, a configuration that would deteriorate the RF performance of the CDN. The actual implementation of the AMN/CDN could look like the diagram in Figure 3. The three resultant load-side impedances: line(s)-to-PE, line-to-line, and common-mode as occurring at the various ports are given in Figure 4. With the addition of the CDN resistors in front of the AMN, a 5-Ω resistor needs to be added in series with each of the 50-Ω measurement ports of the AMN to maintain the 50-Ω impedance between line-to-PE.

As can be seen from Figure 4, all impedance requirements can be met with a single network. Each “essential half”—the AMN and the CDN—carry out measurements in a certain frequency range. The AMN measures between 10 kHz and 30 MHz while the CDN measures between 150 kHz to 230 MHz. The asymmetrical disturbance voltages shall be measured at the nodes: V2 and V5 (Figure 3); and the common-mode disturbance can be measured at node V8. The 50-Ω resistors at these measurement positions represent the load impedance—i.e., input impedance of the EMI receiver, selective voltmeter, or spectrum analyzer. Because of the 5-Ω series resistance with the asymmetric voltage measurement nodes, 1 dB (10 percent) of correction should be introduced:

Additionally, the common-mode disturbance voltage shall be measured for which the RF emission requirements as defined for the telecom terminals4 shall be used.
When the measurement setup as described in the various standards of IEC CISPR and their equivalent European Norms are adapted accordingly, the measurement uncertainty can be reduced from about 22 dB, for common-mode related disturbances down to a maximum of a few decibels. The 6-dB asymmetrical measurement uncertainty can be further reduced by specifying a mains cable that satisfies the line-to-PE and line-to-line characteristic impedances of 50 Ω, (resp. 100 Ω) (Figure 2). The 150-Ω common-mode characteristic impedance can be met by stipulating that the mains cable should be routed at 30 mm above the GRP, rather than the 0.4 meter height that is now used. The device/ appliance shall be placed on an insulating support at a height of 0.1 meter i.s.o. 1.1 meter above the GRP.5

- Table 2. RF emission levels, common-mode mains voltage.
Conclusions
At present, the AMN measurement method as described in IEC/CISPR 16 and used with various conducted RF emission tests (IEC CISPR/European Norms) leads, even when using all measurement uncertainty calculations, to non-acceptable uncertainties in the measurement of common-mode related disturbances, which can be as much as 22 dB or even more.
The lack of a pre-described mains cable cross-section to satisfy the various characteristic impedance constraints with AMN measurements leads to another 6 dB of uncertainty (Figure 2).
If the description of the AMN is changed to that of an AMN/CDN to satisfy the mains supply wall outlet impedance requirements, the conducted RF emission standards in all of the EMC standards must be adapted accordingly. The mains cable between the devices/appliances and the AMN/CDN must be routed 30 mm above the ground reference plane. The ground reference plane needs to be grounded to the AMN/CDN. The devices/appliances shall be placed on an insulation support 0.1 meter in height, similar to that described in IEC 61000-4-6.6
All the AMN as well as the CDN related RF emission measurements can be carried out in their frequency band of application; AMN: 10 kHz to 30 MHz, CDN: 150 kHz to 80 (230) MHz with a single network and one common test setup.
The AMN/CDN is also recommended to be used with radiated EMC tests; RF emission, RF immunity4, 5 to stabilize (= improve reproducibility, i.e., reduce measurement uncertainty) caused by the mains cable routing.When introducing the AMN/CDN, the measurement setup used with RF emission as well as RF immunity test setups can be fully harmonized.
Also secondary lines connected to the devices/appliances shall be terminated in common mode during the mains disturbance measurement test by an ISN/CDN.
References
- IEC CISPR 16—Specification for radio disturbance and immunity measuring apparatus and methods
- IEC CISPR 20—Sound and television broadcast receivers and associated equipment – Immunity characteristics – Limits and methods of measurement
- IEC 61000-4-4—Testing and measurement techniques – Electrical fast transient/burst immunity test
- IEC CISPR 22—Information technology equipment – Radio disturbance characteristics – Limits and methods of measurement
- IEC 61000-4-3—Testing and measurement techniques – Radiated, radiofrequency, electromagnetic field immunity test
- IEC 61000-4-6—Testing and measurement techniques – Immunity to conducted disturbances, induced by radio-frequency fields
- Loes van Wershoven, “The Effect of Cable Geometry on the Reproducibility of EMC Measurements. Proceedings of IEEE International Symposium on EMC. Seattle: 1999.
- H. Krattenmacher and A. Schwab, “Measurement of Conducted Emissions—Possible Sources for Measurement Uncertainties.” Proceedings of IEEE International Symposium on EMC. Seattle: 1999.
- Marcos Quílez, Jordi Escoda, and Ferran Silva. “Analyzing the Repeatability of Conducted-Emissions Measurements.” www.ce-mag.com/archive/01/Spring/Silva.html.
Mart Coenen has over 28 years’ experience in EMC in various fields and has published many papers and publications. He has been actively involved in international EMC standardization since 1988 and last year was awarded with the IEC 1906. He is the former project leader of the standards: IEC 61000-4-6 and IEC 610004-2 but moved his focus towards EMC in integrated circuits. At present he is convenor of IEC TC47A/WG9 and member of WG2.In addition to his career with Philips, he is co-founder of the Dutch EMC/ESD Society and part-time lecturer of Post Academic EMC courses. Aside from these activities, he owns a private EMC consulting company.