The Question
What is the required bonding and grounding requirements to achieve compliance with the various electromagnetic compatibility (EMC) standards associated with electronic equipment?
I received this question a few days ago and quickly realized that a quick and easy answer was not feasible since I virtually had no knowledge of what kind of equipment types were involved, and no information on the application. The more I thought about the question, the complexity of considerations grew and I realized that the approach was dependent on the situation.
Most of us in the EMC community have been brought up with the common concept of 2.5 milliohms, which has been drilled into us without the rest of the statement regarding where that magic number applies. Many believe that to reach compliance we must get to less than 2.5 milliohms from any point to the installation connection. We have been pushed into that goal for the test configuration without regard for the reality of the equipment bonding when installed, so the test configuration fails to conform to what is expected. Even worse is that when issues arise in fielded items, the solution is to fix the installation.
We must realize that compliance testing should approximate a realistic installation to determine if things need to change. MIL-STD-461G made a large step in that direction by specifying that various bonding measurements be made for the test configuration to validate that the bonding was representative of the installation. Values were NOT established (except for ground plane to enclosure and LISN to ground plane) to support confirmation that the installation attributes have been simulated. Even with this change, the age-old target of 2.5 milliohms over multiple connections is often pushed by experienced authorities because of the way they were taught.
The Goal
The goal for grounding is to provide an electrical connection to a common reference (normally considered to be earth) to reduce potential differences that allow shock hazards and noise voltage to appear. The ground should:
- Establish a conductive path for lightning to protect the facility, people, and equipment.
- Reduce voltage levels on accessible points to a non-hazardous level including fault conditions and lightning discharge events.
- Support noise control by reducing voltage differences between the source and destination of signal circuits.
Many guides provide information on grounding based on the earth as the reference point, and I suppose that is correct when considering facilities. But, how about for aircraft, vehicles, ships, spacecraft, and handheld items? In these cases, ground becomes a simple reference point that we like to believe is 0 volts, when referenced to our body.
T to achieve this 0-volt ground, the resistance between points must have no resistance (or impedance), which is not realistic. Conductors have resistance distributed along the conductor and to add more resistance each conductor junction has resistance, so bonding resistance needs to be minimum to reach the 0-volt potential difference. As we can clearly see, grounding and bonding are directly linked, however, bonding also applies to live circuit connections to prevent voltage drops along the path. Keeping bonding impedance minimum prevents voltage from being developed when and if current flows in the conductor path.
This review is not meant to be a design guide providing details on how much, how long, how big, how tight, and the many other elements that must be determined to establish an efficient workable ground system. Since the ground system serves many purposes, I just want to look at things to be considered and how they affect other goals of the ground system. An effective ground system meeting the defined goals must be designed, not left to chance nor just attaching a conductor to make a ground connection.
Electrical Safety
Grounding is often used to provide a means of protection from electrical shock hazards that could occur in various fault conditions. Many electrical equipment regulations include the requirement to provide this means of protection although many situations use another means of protection if grounding is not reasonable.
In the situation where hazardous voltages are present, grounding presents an impedance so low that the grounded point cannot have a potential that could cause a human body shock.
What needs to be grounded? Accessible conductors that could become live in a fault condition. For example, a metal equipment cabinet becomes live because a crimp terminal lug allows the wire to fall out from vibration, and the exposed unrestrained wire contacts the cabinet. The equipment stops working because the wire is the primary power lead and the technician going to repair the item becomes the conductor to ground when contacting the chassis if the safety ground is not present. If the safety ground is connected properly, then the circuit breaker feeding the circuit trips, killing power to the equipment.
Let’s examine a little more to uncover the design for this safety ground. Normally a chassis connection point is provided which may be the third wire in an AC power cord that connects to the outlet ground pin. This connection should be assembled in a manner that makes that connection the first bond to the chassis and it is secured independently of other ground connections with anti-rotation hardware (see Figure 1). For example, the third wire in the power cord inlet connector is routed to the chassis protective earth terminal on the chassis and secured without other terminals in that bonding point. This does not imply that other terminals must be excluded, just that they are separately secured. Don’t forget that the crimp terminal lug if used, has double crimp points.
The wire size must be able to conduct the maximum fault current. So how much ampacity is required? If the power cord is permanently attached the third wire must be equal to the phase wire under the presumption that the phase wire will burn open by the time the ground wire burns open. But, more generally, we fuse the phase lead (never fuse the safety ground) to be sure. If the cord is detachable, it must be assumed that the cord could be exchanged with another that may have different gauge wire that originally supplied. In this case, we rely on the facility circuit breaker. It is common to provide a 15- or 20-ampere circuit to ordinary outlets, so the wire gauge needs to support that normal situation. To satisfy that grounding need, a 12 AWG wire is frequently selected for the inlet to the ground stud to meet that ampacity. Remember the color (green/yellow) to meet code.
What are the bonding requirements? Most standards call for a resistance of less than 100 milliohms for the safety connection indicating the resistance between the protective earth terminal and the facility (platform) ground. Let’s examine the path bonding and determine what bonding points would apply for a detachable AC power cord. The terminal indicated in Figure 1 (the ground stud) has a bonding junction to the metal chassis; to the secured terminal lug; the terminal lug has a double crimped junction to the wire; the wire length is about 10 cm to the device AC inlet plug connected to a quick connect lug fitted to the inlet; the inlet bonding pin connects to the power cord which is about 1.8-meters long; to the facility AC outlet terminal. The ground wire path contains six junctions and 1.9-meters of wire. The resistance of 1.9-meters of 14 AWG wire is ~63 milliohms, leaving just under 40 milliohms for the six junctions or about 6 milliohms per bonding point. Not difficult to attain this junction (bond) resistance unless we fail to consider contamination or any loose connections along the path, but it does provide insight on the need for good bonding.
Keep in mind that there are other safety-related bonding points, such as doors, drawer slides, and chassis panels that may need to be assured a ground connection. Notice that I used “assured”—not just an incidental contact that may or may not provide an assured connection to ground.
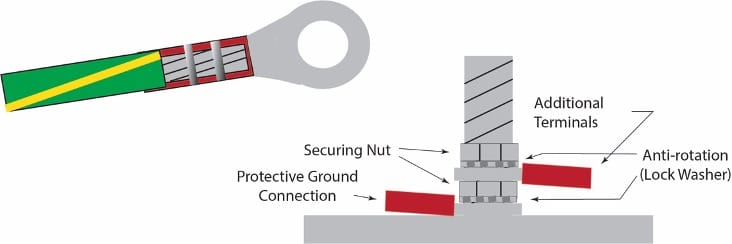
Lightning
Grounding provides a certain degree of safety associated with induced lightning events along with equipment damage protection. The electrical safety requirements described above include lightning transient conduction via the ground system. The lightning induced transient may enter the equipment from external cables, antenna interfaces, or power lines where transient protection components may shunt the lightning current to facility ground or the device chassis and protective ground conductors to the earth connection. Lightning control measures are often incorporated where potential strike currents enter the facility or platform to reduce the risk of the extreme hazards from direct or nearby direct strikes, but the equipment ground system should control the induced transient levels.
As an integral part of the ground, the wiring and bonds are subject to high voltage and current transients associated with induced lightning that may be induced into wiring entering a facility. A transient conducted by a power line (see Figure 2) is coupled into the power distribution within the facility where suppressors limit the voltage and current and the equipment ground system diverts the transient from the equipment. The ground wiring insulation needs to support the lightning-induced high transient voltage developed across the ground connecting system. This capability is evaluated by the required Hi-Pot testing between the external connections and the earthing system where high voltage is applied and is required not to arc to the ground system (note, during Hi-Pot testing transient suppressors are disconnected). Depending on the circuit parameters, the breakdown voltage test levels could be up to 10 kV.
A direct strike to the facility’s lightning protection system provides a down-conductor for the current with a large current for a short transient event. The transient current flowing in the down-conductor may induce current into the facility wiring similar to power line conduction. Relatively speaking these transients are typically limited to a few volts to low kilo-volt levels but the current could be significant if a breakdown occurs. If the down-conductor impedance is high, the induction increases significantly where voltage levels of 100-200 kV appear at the attachment point allowing 40 kA or more transient current through the down-conductor. In addition, the events occur as multi-stroke events with up to 20-strikes occurring within a few milli-seconds. The transient rise time is typically about 2 mS, so the down-conductor inductance becomes a significant factor in the overall impedance associated with frequencies of a few 100s of kHz.
Providing grounding for the lightning protection system requires us to consider the many facets that affect performance. The conductors must have the current handling capability to deal with the near-instantaneous thermal rise related to the ohmic (I2R) power dissipation associated with the event, including the ongoing current and multi-strike events without recovery time. The bonding must prevent high resistance junctions that endure the environmental effects and thermal expansion and contraction that deteriorate the bonds. The transition times indicate that skin depth issues need consideration to manage the overall reactance of the conductor.
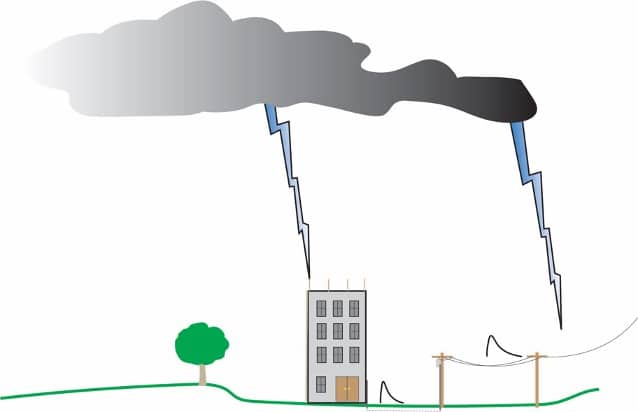
Remember that lightning events stress the capability of the control measures and we frequently are unaware that the various elements have been damaged and are no longer effective. We also have to consider that the lightning attachments do not always select the highest point (see Figure 3).
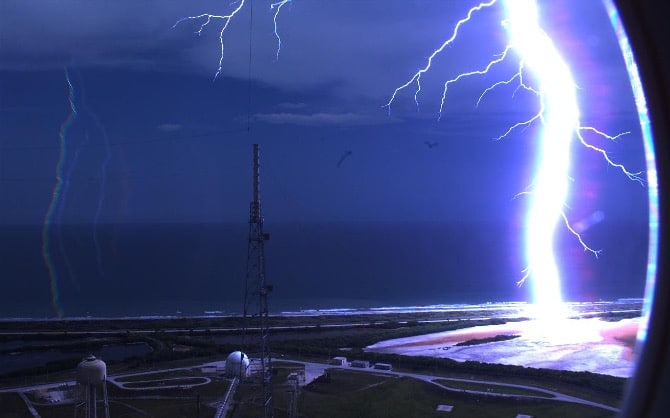
Electromagnetic Compatibility (EMC)
Grounding is normally associated with many of the EMC control measures where the grounding quality is linked with shielding, filter performance, and providing a coupling path for interference to reach sensitive circuits. We are typically coping with higher frequencies than safety or lightning control measures encounter.
At the equipment level, the signals require a return path for the current loop so when a circuit demands current, it must support the return of that current to the source. It is not unusual to provide several “ground” connection types to isolate circuits such as digital from analog. But we cannot forget that we need to get to the common point to terminate the parasitic circuits that are associated with all the circuits. In designing the return and ground the arrangement minimizes the loop area to reduce circuit radiation—plan where the current is to flow without interruption or discontinuities.
Filters often require that a ground path be established to shunt noise current coming into or out of the equipment (see Figure 4). If the bonding impedance (ZB) is high the noise will feed through the line-to-ground capacitance depending on the frequency performance of the circuit. This normally requires that each junction provides a very low resistance and the overall impedance be much less than the feed-through impedance. Note that low impedance is required, so the inductive reactance and parasitic capacitance of the entire path must be considered to meet the goal.
EMC grounding often requires good performance at high frequencies so considering the conductor skin depth becomes a factor in the grounding scheme. The skin effect allows the separation of the signal return from shielding in a coaxial cable where the signal return current is on the inner surface and noise current flows on the outer surface to the coaxial shell termination as indicated in Figure 5. The skin depth effect can carry into selecting a ground strap where we often choose straps with a low length-width ratio to reduce the inductance. The skin effect allows us to use hollow tubing instead of bars to add flexibility in routing the strap. A flattened tube can perform the function and the normal rounding of corners helps reduce radiation from the conductor. The strap must have sufficient ampacity to handle the current at lower frequencies for safety aspects of the design.
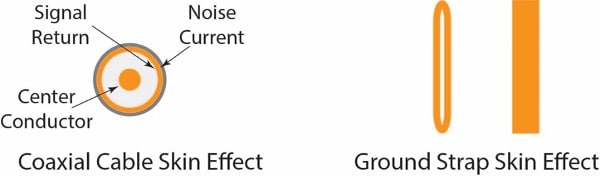
Shields need to be grounded to enhance the shielding effectiveness by providing a radiated field impedance mismatch. An ungrounded shield often acts like an antenna radiating the signals from the surface. For example, a chassis door that is not bonded to the chassis may act in this manner. Placing a wire connection between the door and the chassis may provide a low resistance and meet the safety requirements, but the high frequencies may radiate if the impedance is excessive. Bonding completely around the door frame can mitigate this risk.
I think that mention of the 2.5 milliohm myth should be included here. For years, a requirement for the ground system to have less than 2.5 milliohms resistance. This has been widely construed to mean that any point on the chassis should measure less than 2.5 milliohms to the facility/ground plane. MIL-STD-461G has tried to resolve this misconception but it remains an issue. MIL-STD-461G includes a requirement to measure the ground resistance and provide measurements in the report without defining a specific number. MIL-STD-464C provides some specific resistance measurement requirements, but the only 2.5 milliohms requirement is for an individual faying connection. We need to make sure that the EMC community realize the testing with artificial ground resistance measurements promotes the need for installations to achieve the same resistance. The real goal is to provide grounding resistance/impedance that represent the installation. Then is additional grounding is needed to comply, the installation must be coordinated to provide the required terminations.
Summary
The grounding and bonding must meet all of the required goals—the EMC grounding scheme needs to include the safety requirements and lightning protection as needed. Meeting all of the goals goes beyond just making an electrical connection. Consideration for size, junctions, frequency response, overall impedance, physical constraints, and the installation environment must be part of the design.
I recall a product where compliance had been obtained with a few modifications to the original item during test. To support manufacturing, I had the opportunity to layout a backplane for automated connector placement. Since I was doing a layout anyway, I redid the circuit board ground layout to better support EMC control. No component changes were made, but the update to the ground scheme alone the EMI radiated emissions were reduced by more than 17 dB and the device receiver sensitivity was improved by 10 dB—a major change in the operational performance.
Grounding and bonding should never be left to chance—these factors have a significant impact on performance and compliance. If considered early in the design, controls can be incorporated with a minor cost impact especially when compared to the cost of modifying a mature design.
This review is just an overview of bonding and grounding, details would fill a large text to consider many examples of ways to achieve the goals. Many regulations point to specific elements like the size of the wire, ground rod depth and diameter, and other factors. But, alternate approaches can be used to support other needs if the regulation requirement is also met.
Don’t forget that current flow where directed if the installation is correct. Connecting a shielded enclosure to the facility ground should be accomplished on the wall where current is directed away from sensitive panels. This philosophy applies to the equipment design, route the current where you want it to go.
If you have a topic associated with EMC that you would like to have reviewed, let me know and I will try to place it in the queue for future articles.