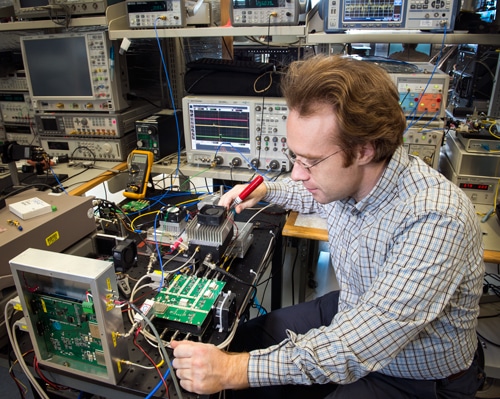
Researchers at NIST’s Physical Measurement Laboratory have created a new, highly-efficient single-photon avalanche diode (SPAD) that is able to detect millions of photons per second while limiting noise.
SPADs are detectors able to amplify the effect of one photon into a signal large enough to quantify and measure, amplifying miniscule electrical “hits” into an exponential “avalanche” of excited electrons whose charge can be easily detected. Such capabilities have made SPADs integral in high-speed quantum communication systems that require navigating massive streams of high-speed photons. In order for the device to detect a discrete photon, electronic signals are applied to the SPAD, alternately raising and lowering its voltage in order to create distinct “gates” of time during which photons can be detected.
However, while SPADs have been integral in secure quantum communication systems – specifically, to locate and isolate individual photons in order to utilize their quantum-mechanical states to create particular encryption keys – their reliability has been questioned. Previous SPAD technology has been especially vulnerable to a phenomenon known as “afterpulsing,” which results when an instance of photon detection causes a second, false pulse to follow. In order to resist these afterpulses, electronic signals are applied to the detector. However, such signals create a great deal of electrical interference, making it more difficult to pinpoint the miniscule electrical pulse generated by an actual photon detection. Because of these interference issues, previous SPADs have been criticized for their imprecision.
Now, researchers at PML have been able to combat such imprecision, maximizing detector performance using a new control system that crucially limits electrical interference by a huge factor – more than -65 dB – by reducing the charge, and time, needed per detection cycle.
“The whole idea is to have as few charges in the material as possible after you have accumulated just enough for a signal,” said Quantum Optics Group Leader Alan Migdall, clarifying that “…reducing the time interval for a factor of two caused almost an order of magnitude reduction in the number of charge carriers in the SPAD.”
In other words, increasing the device’s voltage and amplifying the signal would only increase the probability of afterpulsing or other kinds of electronic interference. By manipulating the electrical signals that dictate the “gates” of each SPAD detection cycle, the researchers were able to produce a much tinier electrical signal, creating a signal pulse consisting of not one, but four “synchronized harmonies.” By minimizing the time and charge needed for each detection cycle, this new kind of SPAD dramatically reduces the possibility of damaging electrical noise.
“This is really a big improvement,” said Joshua Bienfang of the Quantum Measurement Division’s Quantum Optics Group. “Our afterpulsing is roughly an order of magnitude lower than other systems at similar detection efficiencies. The low avalanche-detection threshold has really proven its value. There is a statistical variation in avalanche size, so our ability to detect smaller signals is one of the reasons we see higher detection efficiencies.”
“Extracting their full potential has been a challenge, and that’s where we’ve been focusing our attention,” said Bienfang, on SPADs. “The idea is, ‘Ask not what your SPAD can do for you. Ask what you can do for your SPAD.’ ”
-Melanie Abeygunawardana