Introduction
Although it should be well known that the shielding effectiveness of a cable is severely compromised when it is terminated to ground with pigtail/s many equipment manufacturers still use this technique.
We see in Reference 1 Figure 7.66 and Table 7.4, Figure 7.80, and Figure 7.102 that the shielding effectiveness of a coaxial cable with pigtail terminations may be zero at some frequencies. This is due to the inductive reactance of the pigtail. In a coaxial cable, this reactance is in series with the signal so a voltage is developed across it due to signal current flow, and results in electromagnetic radiation from the cable. In the case of a radiated or conducted susceptibility/immunity test, the incident field induces a voltage in the pigtail, which, in the case of the coaxial cable, is in series with the signal voltage. It was required that the coaxial cables in equipment under test (EUT) be isolated from the chassis at both ends because the shield was connected to signal ground. The cables entered the equipment at both ends of the cable through connectors, and the test currents induced in a radiated or conducted susceptibility test flow into the equipment enclosures resulting in radiation from and current flow on the signal grounds in wires, cables, and PCBs.
The idea that using a triaxial cable with the outer shield connected to chassis and the inner shield isolated from chassis and connected to signal ground, which is equivalent to the coaxial cable, seemed like an ideal solution, except that the connector chosen did not have an EMI backshell, and the only method of terminating the outer shield was pigtails at both ends of the cable. Despite the use of pigtails, it was decided to analyze how effective the triax was in the radiated susceptibility test. Because the shielding effectiveness of a shielded cable is reciprocal, if the induced voltages in the radiated susceptibility test were reduced then so would the current flow on the cable resulting in lower radiated emissions.
Reference 3 describes the tests performed on the triaxial cable with pigtails and a coaxial cable either with the shield connected to the chassis at one end or floating from the chassis at both ends. The measurements in Reference 3 show a lower induced voltage on the triax cable with pigtails from 7-15 dB. This is not due to the anechoic chamber, which is very well damped (Reference 1 page 807, Figures 9.59 and 9.6) and only shows a difference of 3 dB at these frequencies compared to an open area test site. The difference may be due to the presence of the copper pipe and the effect of the anechoic chamber on the transmitting antenna.
The Effectiveness of the Triax Versus the Coax Was Analyzed Based on the Radiated Susceptibility Test Modeled Using the Methods of Moments (MOM) Formulation
In this investigation, the triax outer shield is connected to the EUT and the test equipment at the far end of the cable by pigtail connections. Two pigtail loops were used each 8.26cm long and the wire is 16 AWG. The calculated inductance of the two loops in parallel is 0.0104 uH.
The triax inner shield is not connected to the chassis but used as a signal return. In the case of the coaxial cable test, the shield is terminated to the ground plane with a low impedance.
The current induced down the length of the 3-m-long cable and the current in the terminations at the specified E field is modeled using the 4NEC2D Method Of Moments (MOM) program. The MOM is only valid for an open circuit wire or a wire terminated at one end only (asymmetric connection), but not where the wire is terminated at both ends (symmetric connection). This is not a problem as the currents in the symmetric connection are the same only the resonant frequencies are different e.g 125 MHz symmetrical connection 150 MHz symmetrical. For a wire terminated at one end, the current at the termination end of the cable is maximum and 0 at the far end. Thus, the first resonance for the symmetrical connection is a 1/4 wavelength. For a 3m cable and a 1/4 wavelength, the wavelength is then 4 x 3 m = 12 m, and the frequency is 300/12 = 25 MHz. The next resonance is when the cable is 1 and a 1/4 wavelengths long. 1 and a 1/4 lambda = 3 m and a wavelength is 2.4 m = 125MH.
For the symmetrical connection (either a cable connected to the ground at both ends or isolated from the ground at both ends) resonance is when the voltage at either end is zero. Thus, the first resonance is when the cable is 1/2 wavelength long. A wavelength is 6 m and the frequency is 300/6 = 50 MHz. The model is for a 3 m long cable at a height of 5 cm above a ground plane and 10 cm from the front with an antenna 1 m from the front of the ground plane and 0.5 m above the ground plane.
Figure 1 shows the MOM model.
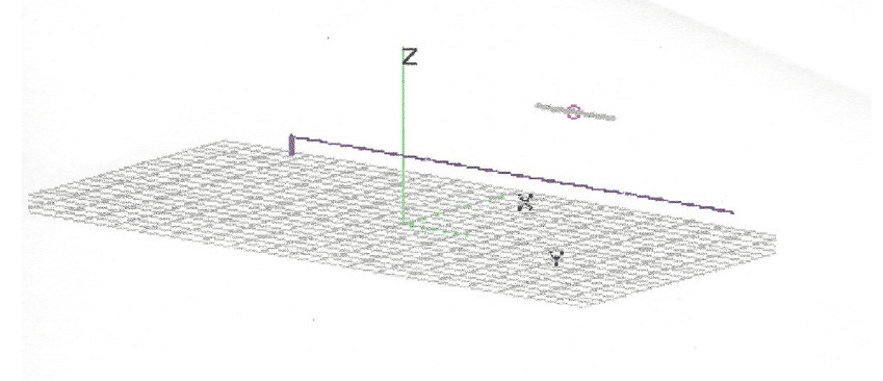
Coaxial Cable
The coaxial cable used is the Alpha wire part no. 9058A, which has a shield optical coverage of 95% but does not specify the shielding effectiveness or the transfer impedance so the coaxial cable transfer impedance was for a typical cable with good optical coverage (this is typically 90-96%) from Reference 1. Although not as good as the RG58 tested in Reference 3, the triax configuration was still better than the RG58 coax in those tests.
We assume that for the coaxial cable with a low impedance termination to the chassis that this termination is outside of the enclosure, typically through a coaxial connector outer EMI backshell. If the coaxial cable is taken inside the enclosure and then connected to signal ground or chassis then the current in a susceptibility test enters the enclosure and will radiate. Likewise, the electromagnetic ambient inside the enclosure will couple to the shield and result in an increase in radiated emissions. With this cable configuration, the shielding effectiveness will not be dependent on the transfer impedance only. Reference 3 shows measurements with this cable configuration.
Comparison of the Voltages Induced Into the Coax Cable, Triax Cable With Pig Tails and Triax Cable With Low Impedance Shield Termination
The triaxial cable used is a Belden 9222 004100. Belden does not provide shielding effectiveness or transfer impedance for this cable and so a sample was procured to test. The transfer impedance is described in the paper, Reference 2, “Measuring the transfer impedance and transfer admittance of a triaxial cable with either a low impedance connection of the outer shield or with pigtail terminations.” Reference 1 describes the line injection test set up and the measured transfer impedances both near end and far end. The triaxial cable has an additional outer braid isolated from the inner braid compared to a single shield for the coaxial. The MOM predicted current flow in the outer shield of the triaxial cable, in the coaxial cable shield, and in the pigtails due to the worst-case 200 V/m radiated susceptibility test is shown in Table 2 at the resonant frequencies. Although 200 V/m was used, this may be scaled according to the requirements. For example, the DO-160 worst-case radiated susceptibility limits are 20 V/m at 125 MHz and 150 V/m at 350 MHz, 1,100 MHz, and 3,000 MHz.
Frequency (MHz) | Coaxial or triaxial cable shield current Corrected
for 200V/m |
Pigtail current Corrected for 200V/m | Pigtail inductance (H) | Pigtail reactance * Ω | Voltage developed across the pigtail ** |
125 | 1.76e-1 | 1.63e-2 | 7e-8 | 55 | 0.896 |
350 | 3.98e-1 | 0.252 | 7e-8 | 154 | 38.8 |
1100 | 2.06e-1 | 0.095 | 7e-8 | 484 | 45.8 |
3000 | 5.4e-2 | 0.008373 | 7e-8 | 1320 | 11 |
Table 2: Current induced in the coaxial cable and triaxial cable shield and the voltage across the pigtail induced by the DO-160 specified 200 V/m RS test levels. * The pigtail reactance is given by 2p x f x pigtail inductance. ** The voltage is the pigtail reactance x the pigtail current.
For low-frequency signals, a signal filter may be used to attenuate the higher frequencies shown in Table 2. Reference 1 describes the design of many types of signal line filters. The E field predicted in the MOM analysis had to be corrected for a test field of 200 V/m at 125 MHz, 350 MHz, 1,100MHz, and 3,000 MHz. The Zt of the triax with and without pigtails was based on measurements made from 1 MHz to 1.1 GHz with the results shown in Reference 2. Table 3 shows the predicted and measured transfer impedance for the coaxial, triaxial with pigtails, and a triaxial with the outer shield connected to the ground with a low impedance connection. The measured transfer impedance of the triaxial cable with a low impedance termination and the pigtail termination is given in Reference 2.
Frequency | Zt of coax (based on published data)
( Ω) |
Zt of triaxial cable with pigtails (from measurements)
( Ω) |
Zt of triaxial cable no pigtails (from measurements)
( Ω) |
125 | 6 | 1.2 | 0.5 |
350 | 15 | 3 | 1 |
1100 | 30 | 6 | 1.2 |
3000 | 36 | 6.7 | 0.2 |
Table 3: The predicted transfer impedance for the coaxial, triaxial with pigtails, and a triaxial with the outer shield connected to the ground with a low impedance connection.
Figure 2 shows the envelope of the highest Vt between the center conductor and shield in the RS test with 200 V/m. In Figure 2 measurement of the coax cable is connected to the chassis at both ends via a low impedance termination due to the voltage developed in the pigtails. The triax with pigtails is better than the predicted coaxial cable because not only is the outer shield connected to the chassis with the pigtails but also the inner shield. When the triax outer shield is also connected to the chassis with a low impedance then the transferred voltage is at its lowest with the exception of the resonances previously mentioned. Measurements on an RG58 cable with the shield connected to the chassis at both ends show a transfer impedance close to the triaxial cable with the outer shield connected to the chassis at both ends, however, that is not how the coaxial cable is terminated in the EUT.
Reference 3 describes radiated susceptibility tests where a comparison is made between the coaxial cable and the triaxial cable in the following configurations:
- Triaxial cable inner shield terminated in 1 MegOhm to chassis
- Triaxial cable inner shield connected with 50 Ohm to chassis
- Triaxial cable center conductor connected with 50 Ohm to chassis
- Triaxial cable center conductor connected with 1 MegOhm to chassis
- Coaxial cable with shield connected to chassis with 1 MegOhm
- Coaxial cable with center conductor connected with a 50 Ohm load and the shield connected to chassis.
In all of these tests, the voltages measured were with the shield of the coax floating from the chassis at the far end and with a 50 Ohm load. Likewise the inner shield of the triax cable. The outer shield of the triax cable was connected to the chassis at both ends with the pigtails described in this report.
The conclusion from the Reference 3 tests is that at most frequencies the triax has a lower voltage induced into it from the test field than the coax.
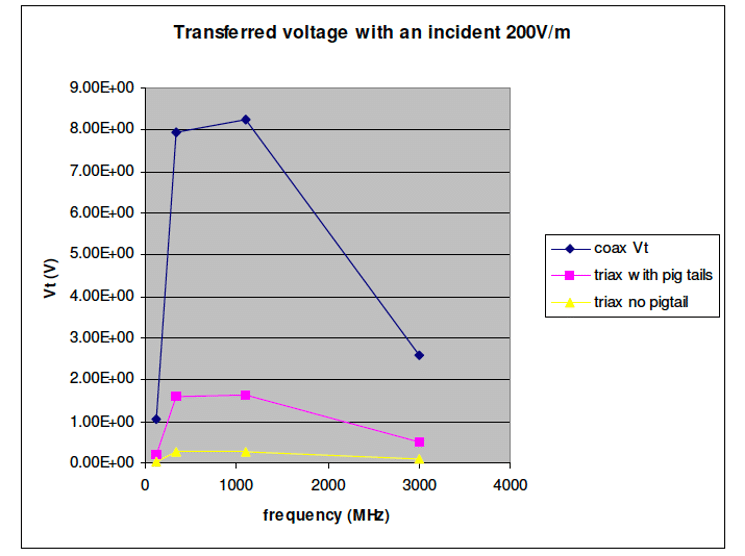
The induced voltages in Figure 2 are shown in Table 4.
Frequency (MHz) | Vt coax, low impedance termination
(V) |
Vt triax with pigtails (V) | Vt triax, low impedance termination
(V) |
125 | 1.05 | 0.211 | 0.035 |
350 | 7.95 | 1.59 | 0.265 |
1100 | 8.24 | 1.65 | 0.275 |
3000 | 2.59 | 0.518 | 0.086 |
Table 4: Vt at 200V/m
The transfer impedance of a cable is Vt divided by the shield current and is due to the mutual inductance between the shield and the inner conductor. Even if the center conductor is an open circuit at both ends Vt appears between the center conductor and the shield as long as current flows on the shield. Vt has a low source impedance and so between a 50 Ohm or an open circuit load between the shield and the center conductor Vt is substantially constant. At lower load impedances Vt does change and this is shown in Figure 3.
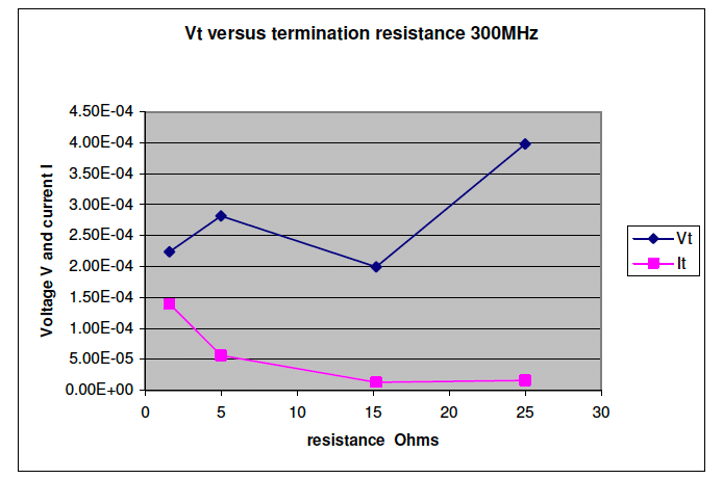
The transferred voltage of the triaxial cable will be lower than the coax cable with low impedance termination to the chassis as shown in Figure 2.
The transferred voltage is due to the transfer impedance between the triaxial cable outer shield and the inner shield and will be lower than the coax cable where the shield is exposed to the incident E field. The transferred voltage will be the same regardless of whether there is high impedance termination between the inner shield and the inner conductor or a 50-Ohm termination, as Vt source impedance is low, and so no appreciable difference is seen between 50 Ohm and an open circuit load, it is only when the load impedance is much lower than 50 Ohm that Vt is lower as seen in Figure 3.
Transfer Admittance Due to the Pigtail
Strictly speaking, the transfer admittance of a cable is the voltage applied to the cable resulting in a current inside the cable coupled through the apertures in the cable. Here it is due to the capacitance between the outer shield and center conductor. Normally it is insignificant, however, the pigtails result in a voltage above ground and this is coupled via the shield to center conductor capacitance. The capacitance between the outer shield and inner shield of the Belden 9222004100 triax cable is approximately 1,300 pF for a 3 m length and the capacitance between the inner shield and center conductor is 310 pF. This was derived from measurements. Because these capacitances are in series, the total outer shield to center conductor capacitance is 250 pF and so is lower in the triax than the coax.
The triax inner shield is either left floating in Figure 4, connected to the chassis at one end only in Figure 5, or at both ends in Figure 6. In the case where the inner shield is terminated at both ends, theoretically as the capacitively induced current flows down the length of the cable in both directions and the transfer impedance current flows in one direction only, at low frequency these two currents add at one end and subtract at the other end. At high frequencies where the cable is electrically long, then this is less likely to be true. The RF conducted susceptibility test induces a current into the outer shield of the cable in a similar way to the RS test however the currents in the pigtails are out of phase, unlike the RS test where they are in phase.
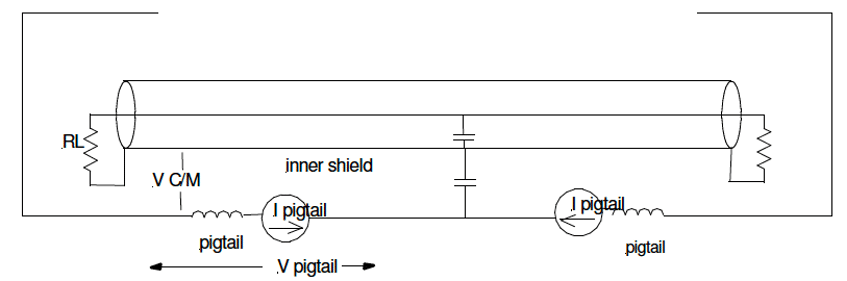
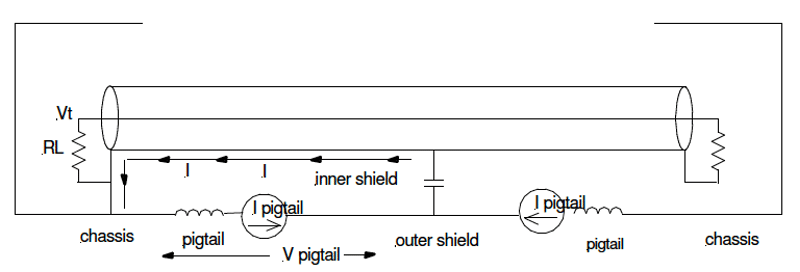
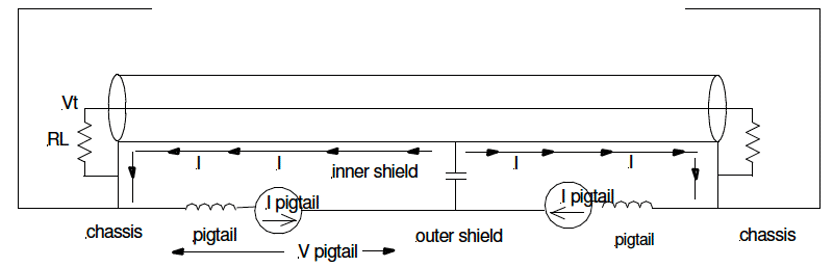
Figure 4 shows that the triaxial inner shield penetrates the enclosures and is not connected to chassis ground and so the C/M voltage should be higher and result in more radiation inside the enclosures. Tests show that this is only true at some frequencies as shown in Figure 9 of Reference 3.
With the inner shield floating, the common-mode voltage on the shield is capacitively coupled to the inner conductor and the differential mode (between the inner shield and inner conductor, as shown in Figure 4), should be zero. Measurements with a 1 MegOhm load show that it is not zero but significantly lower than the C/M as shown in Figure 9 of Reference 3.
Conducted susceptibility and Induced signal susceptibility
ISS Test
In the induced DO-160 signal susceptibility (ISS) test, a voltage is induced in the electric field test at 380 Hz to 412 Hz, and the spike test with pulse repetition rates of 0.2-10 uS. In the electric field test, the triax shield provides a very low impedance and the inductive reactance of the pigtail due to the low frequency is low; thus, the shield provides a very effective attenuation of the field.
Magnetic Field Test
In the magnetic field test at category AC, a field of 18 A/m is incident from 380 Hz to 412 Hz, i.e. 6 A for a 3 m long cable. The low-frequency transfer impedance of a cable is equal to the dc resistance and the current induced is also a function of the terminating impedances. Even in the case of the outer shield of the triax cable terminated with pigtails the inductive reactance of the pigtails is lower than the dc resistance of the cable, which for a 3 m length is 0.047 Ohm. With the center shield floating, the induced current should be close to zero. Consider the case of the coaxial cable where the terminating impedance of the cable shield is much lower than the dc resistance of the cable. The current, from measurements in the test cable, is very approximately 1/2 of the injection current. The induced current in the DO-160 ISS test induces a voltage in the outer shield of the cables = 0.047 Ohms x 6 A/2 = 0.141 V. This voltage appears in series with the signal in a coaxial cable. In the case of the triaxial cable the current in the outer shield induces a current in the inner shield via the mutual inductance of the cable. The mutual inductance is equal to the outer shield inductance and from measurements, this is equal to 2.3μH.
In the magnetic field test, current flows in the triaxial outer shield, and a Vt is seen between the outer and inner shield but it is only when the inner shield is terminated to the chassis at both ends does a current flow in the inner shield. Therefore, the transferred voltage in this test will be lower for the triaxial cable, where the inner shield is floating, than the coaxial cable.
Radiated Emissions
In the radiated emission test, radiated emissions from the coaxial are likely to be higher than the triaxial as the coaxial cable penetrates the enclosure and so the electromagnetic ambient inside the equipment is coupled to the coaxial shield and can result in radiation. As the outer shield of the triax is connected to the chassis via pigtails some current will be induced from the inner shield to the outer shield but this will be lower than in the coax case. The shielding effectiveness of a cable is reciprocal. That is the voltage coupled inside the cable from an external source is lower when the transfer impedance is lower and the shield current flow due to a voltage applied between center conductor and shield, results in a lower level of emissions when the transfer impedance is lower. The current flow on the outside braid of a shield is due to the transferred voltage and the internal shield current. In the case of a solid pipe where the transfer impedance is very low, due to the skin depth effect, all of the current flows on the inside of the pipe and virtually none on the outside. The radiation may be so low as to be below the measured noise level.
Conducted Susceptibility Test
The conducted susceptibility calibration setup is shown in Figure 7 and the injection set up in Figure 8. In the conducted susceptibility injection into the triaxial cable with pigtails, current flows on the outer shield, and a voltage is developed in the pigtails as shown in Table 5.
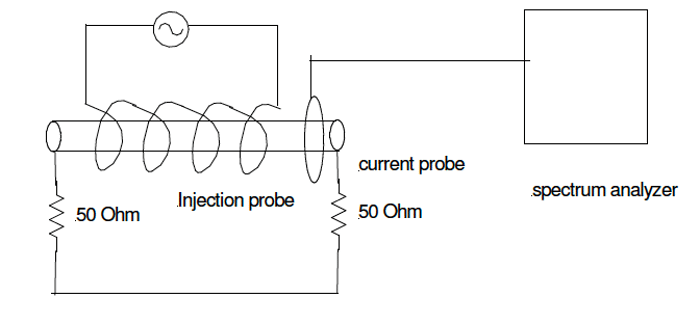
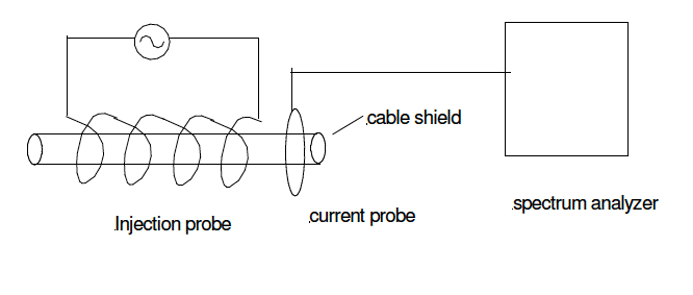
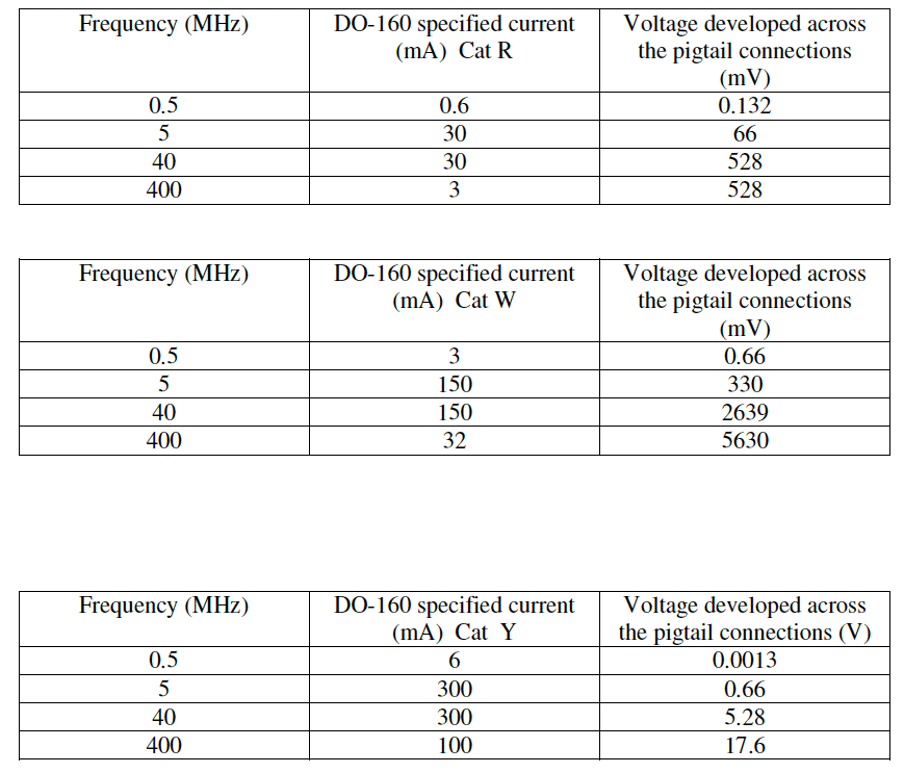
The pigtail voltage is coupled through the outer shield to the inner shield via the 1,300 pF measured capacitance, and the C/M voltage generated by the injection probe appears on the inner shield when it is isolated from chassis. In the case of the coaxial cable, the voltage is the open-circuit voltage generated by the injection probe. This may be lower than the calibration voltage. Thus, for category Y at 300 mA, the calibration voltage is 300 mA x 50 Ohm = 15 V. Measurements on the voltage induced in the inner shield of the triax cable shows the voltage is up to 37 dB lower at low frequencies but equal to the calibration voltage at higher frequencies.
Conclusions
We see from the analyses that EMI voltages induced in the RS test and the ISS tests are all lower using a triax cable versus a coax cable. The voltage induced in the CS test may be lower or equal to the coaxial cable. As the induced voltage in the RS test is lower than the coax so the radiated emissions from the triax will be lower. These conclusions are supported by the measurements described in Reference 3.
References
1 Electromagnetic Compatibility Methods, Analysis, Circuits, and Measurements. Third Edition D. A. Weston. CRC Press 2017. Figure 7.30 Single braid, page 526
2 Measuring the transfer impedance and transfer admittance of a triaxial cable with either a low impedance connection of the outer shield or with pigtail terminations. July 2020.
3 Measuring the shielding effectiveness of a triaxial cable using pigtails to terminate the outer shield t versus a coaxial cable isolated from chassis in a radiated susceptibility test.