EMC system engineers face trade-offs when selecting high power amplifiers for RF immunity testing. During testing, the amplifier must withstand high levels of reflected power. An appreciation of how reflected power affects an amplifier is important if well-informed decisions are to be made. This article uses a simple thought experiment to de-mystify this complex phenomenon and suggests common methods of dealing with high levels of reflected power in amplifiers.
Claims by amplifier manufacturers such as:
“… able to withstand 100% reflected power indefinitely without damage …”,
“… continues to operate into high VSWR …”
abound in the power amplifier market.
Deciphering the actual meaning of these claims can be difficult. Does it mean that the amplifier performance is exactly as if running at full power into a perfect match, or does it simply mean that the amplifier will not be damaged?
Other amplifier manufacturers make absolutely no claim to operation into a severe mismatch. Instead, they tout the virtues of a solution that simply switches the amplifier off as a protective mechanism.
So which way should the system designer go? A better appreciation of how reflected power affects an amplifier helps a designer to make an objective decision. To gain a better appreciation of the problem, let’s conduct a simple thought experiment and then look at how various amplifier designs cope with high levels of reflected power.
SETTING UP THE EXPERIMENT
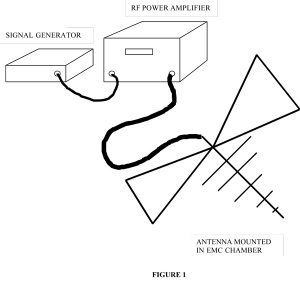
Step 1
Imagine a 200-W, 80- to 1000-MHz RF power amplifier running in a RF immunity test system as shown in Figure 1. For simplicity, the EMC chamber, the PC, and the other system components are not shown.
Step 2
Switch off the signal generator so that the amplifier has no RF input, but leave the amplifier switched on.

Step 3
Substitute the antenna for another identical RF power amplifier and signal generator as shown in Figure 2. This second amplifier is used to inject RF power into the output of the first amplifier.
Step 4
Switch on the second amplifier and signal generator.
We now have a mental construct of a situation in which the effects of reflected power on a RF power amplifier can be considered in isolation.
CONDUCTING THE THOUGHT EXPERIMENT
Imagine the injected RF power entering the first amplifier output connector. It will enter with ease since the RF output path allows RF signals to pass equally well in either direction.
Now imagine the RF power signal reaching and appearing across the amplifier output transistors. The amplifier is still switched on so the transistors have a constant stream of electrons flowing through them (the transistor bias current). Stand at the edge and watch the stream of electrons passing by. What will be the effect of the rapidly varying field caused by the RF power signal? Well, when the field is positive, the electrons will be attracted towards it; and when the field is negative, the electrons will be repelled by it. Obviously, the previously constant current stream will increase and decrease in response to the polarity of the RF signal. The greater the RF field, the greater the change in the current flow.
With the effect of the reflected power signal isolated, let’s bring in the forward power signal.
Step 5
Set the frequency of the first signal generator to that of the second generator. Switch on the first signal generator so that the first amplifier is producing forward power.
Imagine the RF input signal passing through the first stages of the amplifier and reaching the input of the amplifier output transistors. True to their purpose, these RF power transistors will attempt to produce a larger version of the RF input signal by changing their current flow in response to the input signal.
What will the stream of electrons do in response to the two competing attempts to change its flow? Clearly, there will be some type of “tug of war.” Depending on the relative phase, sometimes both signals will try to increase the flow; sometimes both will try to decrease the flow. Sometimes, one signal will try to increase the flow, while the other signal tries to decrease it. In this opposition, the “winner” will be the signal that dominates the other in terms of power. If the forward power is 100 W, and the injected power is 1 W, the effect on the amplifier output signal will be negligible, and only the effect of the forward signal will be observed. However, what happens if the forward power and the injected power are more closely matched?
In exploring this question, let”s replace the injected power source with the more common scenario of power being reflected back into the amplifier output connector.

Step 6
Switch off both signal sources, and replace the second amplifier with a poor match (one that reflects much of the power reaching it) as shown in Figure 3.
Step 7
Switch the first signal generator on again. Follow the forward power leaving the amplifier output connector; watch it hitting the poor match. Observe much of it bouncing back along the cable into the output connector. Also, observe that as the forward power is increased, there is a corresponding increase in reflected power. Changing the frequency controls the relative phase of the reflected power at the output transistors. By changing the power and frequency of the signal generator, we can replicate any of our previous observations, except those that resulted from the reflected power being greater than the forward power. The reflected power is always less than or equal to the forward power.
REAL WORLD OBSERVATIONS
Let”s put our thought experiment aside and look at practical results which show the effect of reflected power in an actual amplifier. Figure 4 shows actual swept power curves for a RF amplifier operating into a good match and shows the same amplifier working into a poor match. The frequency has been selected so that the forward and reflected powers are opposing each other at the output transistors.
Look at the top trace first. This shows the gain of the RF amplifier operating into a good match with little or no reflected power. At low input powers, the gain is fairly constant (trace fairly horizontal); and then, like all amplifiers, gain compression occurs (the gain trace drops) as the power is increased.
Now, look at the lower trace. This shows the gain of the RF amplifier operating into a poor match with 63% of the forward power reflected back. The basic shape is the same as the upper trace, but for identical power sweep conditions, the gain has dropped by 2 dB. What does this mean? Well, for any input power level, the gain will be lower than when the amplifier is operating into a good match. Also, the amplifier goes into compression at a lower power level. The 2-dB reduction means that the power output has dropped by more than a third. The consequence is that the RF amplifier behaves as though it were a power amplifier of much lower power. For example, a 200-W amplifier will behave like a 126-W amplifier. If the system design requires 200 W to guarantee acceptable distortion, then clearly this requirement is no longer achievable. Higher levels of reflected power will only make matters worse.
So what can be done to get around this degradation in amplifier performance? Let”s look, in turn, at the available options.
ALC
Automatic Level Control (ALC) is a mechanism for maintaining the forward power of an amplifier at a preset power level, despite changes to the input power or changes in load conditions. For low to medium levels of reflected power, it is perfectly feasible for the ALC circuit to hold the output power constant. However, when high levels of reflected power start to cause significant deterioration in gain, a point is reached, beyond which the ALC efforts to restore the gain are futile, since any attempt to increase the forward power results in a corresponding increase in reflected power. Most amplifier types, whether Class A or Class AB offer ALC.
Absorbing the Reflected Power
Placing a high power attenuator on the output of the amplifier will reduce the reflected power entering the amplifier. For example, if the attenuator reduces the output power of a 100-W amplifier to 50 W, then even in the worst case, in which 100% of the power is reflected back, the reflected power has to pass through the attenuator again; and the reflected power is reduced to 25 W. Thus, the amplifier is never subjected to more than 25 W of reflected power. This sounds great, but throwing away half of the forward power capability of a RF power amplifier as waste heat is an option almost never taken.
Limiting the Current Available to the Output Transistors
The current flowing through the transistors comes from a DC power supply. Limiting the amount of current supplied prevents the flow of excess current which, in turn, prevents damage by over-dissipation. Still, this protection method cannot correct the loss in amplifier gain caused by reflected power.
Diverting the Reflected Power
There is a component called a quadrature hybrid coupler that diverts reflected power away from the output transistors. This component is used extensively in the power amplifier world, but unfortunately, its bandwidth is limited to just over two octaves. An octave is a doubling of the frequency. For instance, these components are found in amplifiers covering the 500- to 1000-MHz range, the 1- to 2-GHz range, the 2- to 4-GHz range, etc. In fact, these hybrid couplers are what make microwave amplifiers so tolerant of reflected power. However, count the octaves in an 80- to 1000-MHz RF power amplifier as currently used in RF immunity testing:
- 80 to 160 MHz (first octave)
- 160 to 320 MHz (second octave)
- 320 to 640 MHz (third octave)
- 640 to 1000 MHz (just over half an octave).
There are more than three-and-a half octaves, so a quadrature hybrid coupler cannot be used to divert the reflected power away from the output transistors.
Auto-Biasing
Often used in Class A amplifiers and sometimes called “true Class A,” autobiasing is an old, time-honored way of maintaining the bias current in the power transistors at a constant level, despite changes in the power being delivered to the load. The primary purpose of auto-biasing is to provide optimum linearity over a wide range of output power. It also plays a protective role in clamping the average current through the output transistors when high values of reflected power try to push the current to a level that would destroy the transistors. Class A amplifiers are able to dissipate more heat than Class AB amplifiers; but, just as in the case of ALC, the auto-bias cannot correct the reduction in amplifier performance at all levels of reflected power. There is a point above which the mechanism is unable to compensate for the changes caused by high reflected power. The function of the auto-biasing circuit then becomes protective by “clamping,” similar to foldback, which is described next.The plot shows actual swept power curves for a RF amplifier operating into a good match and the same amplifier working into a poor match. The forward power is monitored with a directional coupler. During a power sweep test, the frequency is fixed, and the input power to the amplifier is swept from a low value to a higher value. The output plot of the test shows the ratio of the output power of the amplifier to the input power, i.e., the gain of the amplifier.
Foldback
With foldback, the level of reflected power is monitored, and when a certain threshold is exceeded, the forward power is reduced to keep the reflected power below the level that would destroy the output transistors through excess current. Well designed foldback mechanisms do not begin to limit until the reflected power gets close to a level where distortion or damage may occur. Usually used in Class AB amplifiers to prevent destruction of the output transistors by over-dissipation, it allows the amplifier to continue operating into high VSWR loads. As with auto-bias, the protection mechanism also allows the amplifier to operate into high VSWR loads, but neither mechanism prevents a reduction in amplifier performance in severe VSWR situations.
High VSWR Trip
With a high VSWR trip, the reflected power is monitored and the amplifier is disabled (tripped) when a certain threshold is exceeded. Often used in Class AB amplifiers, it serves as a protection against damage caused by excess reflected power. The basic approach here is simply to switch off the amplifier and to send an alarm signal to the user. See Figure 5 for a plot showing high VSWR trip operation. Part of the rationale here is that the testing ought to discontinue anyway since the test results could well be invalid. This high VSWR trip method is also utilized with great success in TWT amplifiers used in Military and Automotive RF immunity testing. A question comes to mind. Why are TWTA and Class AB amplifier manufacturers not inundated with complaints of ‘nuisance trips’? Well, it is a fact that test runs where an amplifier sees a perfect load with no mismatch are rare. It is equally true that test runs where 100% reflected power occurs are rare. In the vast majority of test runs, reflected power levels will occur at about 10 to 30% of the forward power level. There will be occasions when 30 to 50 percent reflected power occurs, and there will be rare occasions when 50 to 100 percent reflected power occurs. Therefore, ‘nuisance trips’ are rare. More often, when a trip does occur, something has gone terribly wrong in the test setup.
CONCLUSION
It is a pity that diverting reflected power away from the output transistors is not an option here, as this is the ideal solution. An amplifier running at full power into severe mismatch simply does not perform at the same level as when working into a perfect match.
There are two basic types of amplifier offered in the marketplace, Class A and Class AB. Both types are used with great success in RF Immunity Testing. Class A amplifiers offer greater dissipation ability and for protection, usually come with auto-biasing or power supply current limiting or both. Class AB amplifiers cost less, are more efficient, and usually come with high VSWR trip or foldback. Neither amplifier type comes with a magic solution that deals fully with all high VSWR issues, so the risk of invalid test results has to be factored into any selection decision.
Returning to the thought experiment, satisfy yourself that no matter what the phase of the high level of reflected power, none of the protection mechanisms described can correct a change caused by the ‘tug of war.’
On the bright side, you are now in a position to make a more informed choice as regards coping with reflected power and can discern what some marketing statements imply and what they actually mean.
Thomas Mullineaux holds a Bachelor of Engineering degree from Portsmouth University (England) and has 15 years’ experience in leading the design and manufacture of RF and Microwave power amplifiers.