Researchers at the University of Michigan have created a stretchy conductive material that could one day find use in medical implants and flexible display screens and batteries. Created from gold nanoparticles and polyurethane, the material retains its conductivity even when stretched to several times its original length.
Using an electron microscope to examine the effects of tension on the conductivity of two versions of the material, the research team determined that while the nanoparticles started out dispersed throughout the material, under strain they aligned into chain structures that electrons could flow through. The nanoparticles returned to their original position after the stress was released.
“As we stretch[ed], they rearrange[d] themselves to maintain the conductivity, and this is the reason why we got the amazing combination of stretchability and electrical conductivity,” Yoonseob Kim, first author of the study published in Nature and a graduate student in the Kotov lab in chemical engineering, said.
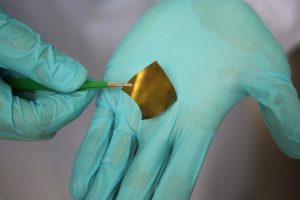
Of the two versions of the material—one built in alternating layers; the other created by filtering a mixed polyurethane and nanoparticle liquid— the layer-by-layer design was deemed to be more conductive, while the material created using the filtered method was considered the more bendable of the two. Without stretching, the layer-by-layer material exhibited an electrical conductance of 11,000 Siemens per centimeter (S/cm)—on par with mercury—while the filtered material had a conductance of 1,800 S/cm, similar to good plastic conductors. When the layer-by-layer material was stretched to its breaking point— more than twice its original length— the team identified an electrical conductance of at 2,400. The filtered material in contrast exhibited an electrical conductance of 35 S/cm when stretched to a maximum 5.8 times its original length.
Kim and co-author Nicholas Kotov, the Joseph B. and Florence V. Cejka Professor of Engineering, believe the ideal use for their flexible material is as electrodes for medical applications. Electrodes that move like human tissue could better avoid damaging the cells, replacing rigid electrodes used in current treatments that create device-inhibiting scar tissue. The material could be particularly useful in brain implants, as well as in flexible electronic displays and in the joints of “lifelike” robots.
“They can alleviate a lot of diseases—for instance, severe depression, Alzheimer’s disease and Parkinson’s disease,” he said. “They can also serve as a part of artificial limbs and other prosthetic devices controlled by the brain.”
Kotov’s team is now exploring various nanoparticle fillers for stretchable electronics, including less expensive metals and semiconductors, on the basis that other materials, such as semiconductors, may also be able to stretch because of the believed universal chain-forming tendency of nanoparticles.