This article has been translated from Dutch to English. It can be found in its original language in the 2014 Europe EMC Guide.
Mart Coenen, Owner, EMCMCC, the Netherlands
The power grid is intentionally meant to distribute electrical energy at the mains frequency, as produced by the electricity generating plants, towards the end-users. The three-phase low-voltage distribution network can be utilized more efficiently when a power factor of 1 is achieved and no harmonic distortion is superimposed. Due to an increase of self-generated energy and the near to saturation increase of switching electronics, the loading of the mains distribution network is heavily affected. These same mains distribution networks are still considered for power line communication in two frequency bands: the lower one, 10 kHz – 150 kHz (EN50065), and the one above from 1,6 MHz to 30 MHz e.g. HomePlug, IEEE1901.
POWER MAINS QUALITY
Power mains quality determines the ‘fitness’ of electrical mains distribution network to the end-user’s devices. Synchronization of the voltage, frequency and phase allows electrical systems to function in their intended manner without significant loss of performance or life. The term power quality is used to describe electric power that drives an electric or electronic load and that enables the load to function properly. Without the proper power, an electrical device (or load) may malfunction, fail prematurely or may not operate at all. There are many ways in which electric power can be of poor quality and many more causes of such poor quality power. By the end-users, it is however generally believed that electrical power is provided 24/7 and capable to deliver the amount of electric power as expected.
The electric power industry comprises electricity generation (AC power), electric power transmission and ultimately electricity distribution through an electricity meter located at the premises of the end-user. The electric power then moves through the mains distribution wiring installation of the end-user until it reaches a load. The complexity of the electric power system to transport electric energy from the point of production to the point of consumption is now combined with variations of the weather: PV (photo voltaic) and wind generation, demand and other factors provide many opportunities for the quality of supply to be compromised or at least be affected.
While ‘power quality’ is a convenient term for many, it is the quality of the voltage – rather than power or electric current – that is actually described by the term. Power is simply the flow of energy and the current demanded by a load and that has become largely uncontrollable.
Ideally, the AC mains voltage is supplied by an energy generating utility as a sinusoidal signal source having an amplitude and frequency given by national standards with a source impedance of zero ohms for all frequencies. The real-life mains power source is non-ideal and generally can deviate in at least the following ways:
- Variations in the peak or the RMS voltage are both important to different types of equipment. When the RMS voltage exceeds the nominal voltage by 10 to 80% for ½ a mains cycle to 1 minute, the event is called a ‘swell’. A ‘sag’ is the opposite situation: the RMS voltage is below the nominal voltage by 10 to 90% for ½ a mains cycle to 1 minute. However, in the USA it is common for transient dips or dropouts to be called ‘sags’.
- Random or repetitive variations in the RMS voltage between 90 and 110% of nominal can produce a phenomenon known as “flicker” in lighting equipment. Flicker is rapid visible changes of incandescence light level. Definition of the characteristics of voltage fluctuations that produce objectionable light flicker has been the subject of ongoing research. With solid-state light sources this phenomena is hardly occurring.
- Abrupt, very short-term increases in voltage, are called ‘spikes’, ‘impulses’, or ‘surges’, generally caused by large capacitive loadings turned-on, by large inductive loads being turned off, or even more severely by direct or in-direct effects from lightning.
- ‘Under-voltage’ occurs when the nominal voltage drops below 90% for more than 1 minute. The term ‘brownout’ is an apt description for voltage drops somewhere between full power (bright lights) and a blackout (no power – no light). It comes from the noticeable to significant dimming of regular incandescent lights, during system faults or overloading etc., when insufficient power is available to achieve full brightness in (usually) domestic lighting. This term is in common usage has no formal definition but is commonly used to describe a reduction in system voltage by the utility or system operator to decrease demand or to increase system operating margins.
- ‘Overvoltage’ occurs when the nominal voltage rises above 110% for more than 1 minute.
- Variations in the fundamental mains frequency, typically within ± 2 % of its nominal value. Variations of the number of mains period per day is restricted due to the common use of synchronous clocks derived from the mains frequency
- Variations in the wave shape – usually described as interrelated harmonics and harmonic distortion resulting from saturation effects in the mains transformers caused by AC/DC rectifiers as these switch at twice the mains frequency (single phase application) at the top of the voltage amplitude.
- There are many more causes of harmonic and interharmonic distortion than just magnetic saturation – for example fluorescent lamps with magnetic or solid-state ballasts can cause due to the switching frequencies that are used. In general, problems are being caused by the connection of all switching electronic loads and switching electronic sources to the grid. Every item of consumer electronics seems to come with a plug-in battery charger which consumes little power but there are huge numbers of them so their overall effect can be large. Frequency inverters (whether driving loads such as variable-speed AC-motors, or synthesizing mains frequency from an alternative power source such as a PV or wind turbine) create other noise frequencies below 150 kHz that also distort the waveform and are called interharmonics because they are not (phase-)locked to the mains frequency.
- Nonzero low-frequency complex impedance: from the medium- to low-voltage power transformer and the wiring impedance towards the end-user’s devices (when a load draws more power, the effective voltage across the load impedance will drop).
- Nonzero high-frequency impedance: when a load demands a large amount of instant current or it stops demanding it suddenly, there will be a dip or spike in the voltage due to the inductances in the power supply line. Also with continuous operated switching loads: UPS, PWM, solid-state lighting controls, etc. the mains impedance is expected to be low but can be high due mains distribution network resonances occurring.
- Active power factor correction. By means of an active power factor controller, the total mains current drawn by a group of loads is measured and capacitor banks are switched on or off in parallel to such a load in such a way that the total power factor at the power transformer remains as close as possible to unity.
As the power mains distribution network constitute several mains wires in parallel and/or branched and already distributed within an end-user building or premises, these mains wires, running in parallel, can also be used as transmission lines. While assuming a formal use of the mains distribution network by the mains harmonic signals, extended by a few harmonics: ≤ 40th harmonic, the higher frequencies ≥ 10 kHz were expected to be free to be utilize for power line communication purposes.
POWER MAINS POLLUTION AND MAINS IMPEDANCE
Both public as well as industrial mains distribution networks are heavily affected by all kind of switching electronics which, for the sake of RF emission compliance, are provided with mains filters having large (X-type) capacitors at their mains side. The intermediate frequency range, between the 40th harmonic of the mains frequency: ~ 2 kHz and 150 kHz, where the EMC requirements start to apply, is presently undefined. On the conducted RF emission side, no requirements are under development, apart from the RF emission requirements which do apply from 9 kHz onwards for lighting appliances: IEC/EN 55015. As such, active infeed convertors (AIC), uninterruptable power supplies (UPS), pulse width modulated (PWM) AC/AC and AC/DC converters and motor drives and other switching supplies, regulators and controllers are unbounded w.r.t. their conducted RF emission and levels up to 30 Volts have been measured in that frequency range. In the latter case, it is unclear against what mains impedance this interference voltage has been measured as it could have coincided with or resulted from a mains impedance resonance.
The mains filter X-type capacitors block i.e. short-circuit RF-wise the transmission line properties of the mains distribution network as required for power mains communication (PLC). In combination with the effective secondary inductance of the mid-to-low voltage mains power transformer as well as the mains distribution network cabling in-between, steep circuit resonances will occur from several hundred Hz onwards. In case active power correction is used by switching capacitor banks in parallel to all three phases of the mains wires, the effective impedance at the secondary side of the transformer i.e. mains ‘source’ side can change from inductive to capacitive, at least for the higher frequencies.
In IEC 61000-3-3 values for the mains impedance at 50/60 Hz are given for mains distribution networks with a loading of 16 ampere/phase maximum. The values: 0,24 + j 0,15 Ω are given for the phase wires and the impedance for the neutral wire is slightly less: 0,16 + j 0,10 Ω as in a TN-S system, the neutral wire is connected to the PE terminal at the power transformer. This results in an effective secondary side transformer stray inductance of 400 – 500 µH for the phase wires to PE. With higher power transformers, the resistance part of the impedance will decrease as where the inductance remains given by the stray inductance of the power transformer and some wiring in-between. When one connects a 100(v3), 10(v6), 1(v9) µF or no(v12) capacitance in parallel to such an AC source, the impedances (20•log10|Z|) as function of frequency are given in Figure 1.
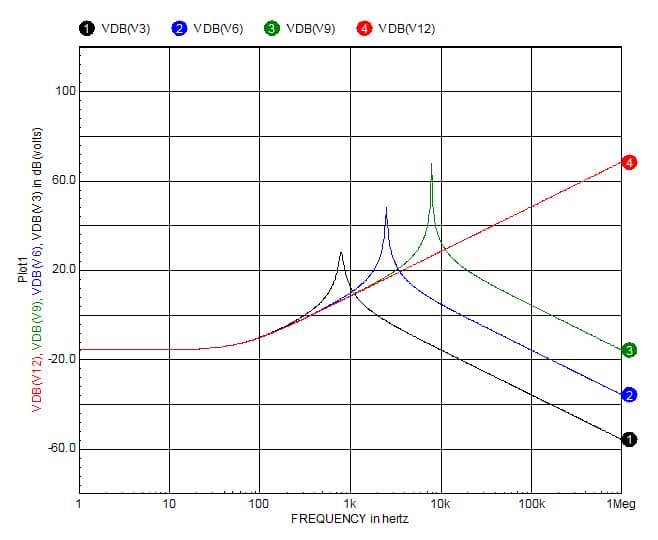
As can be seen from Figure 1, steep impedance resonances from the mains source side may occur from less than 1 kHz onwards. Furthermore, the AC mains source impedance declines above the parallel resonance due to the ideal power factor correction (PFC) capacitor applied. With standard mains distribution network installations, the mains signal is branched from the mid-to-low voltage transformer to the various (building) loads where each and every load has its own complex impedance as function of frequency and branches can be several meters to kilometers long.
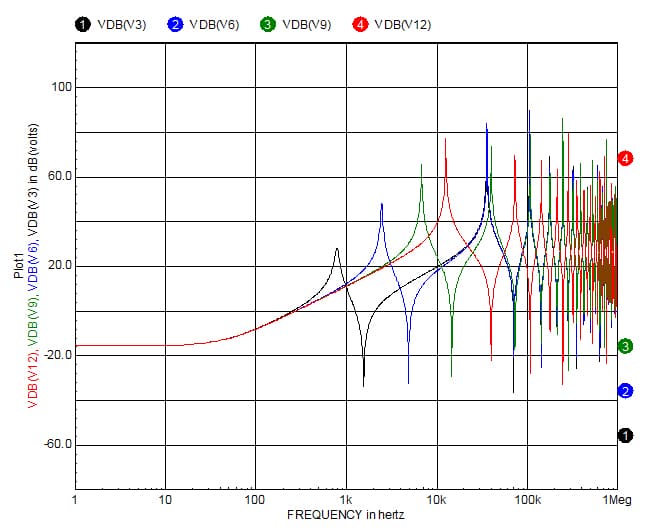
As can be seen from Figure 2, the amount of resonances occurring at the end of a shielded power cable with a characteristic impedance of 20 Ω and being 1 km long is annoying as many frequencies can’t pass through the power distribution network as a result, unless local impedance separation is applied. This simple but realistic example shows that using PFC capacitors prevents i.e. rules out the use of PLC.
From an RF transmission line point of view, the differential characteristic impedance of an in-house or in-building mains distribution network is between 20 and 150 Ω (= 26 to 44 dBΩ). This is determined by the cross-sectional geometry of the mains wiring (max. 5 wires in a hose) versus their environment. Using shielded VMvKas power cables will yield the low impedances: 20 , as where two or three 2,5 mm2 individual wires in a 5/8” PVC tube will yield a high impedance: 150 . An advantage with the shielded power cables is the low RF-losses that occur due to the lack of any radiation resistance losses. The drawback is the lack of any RF-damping and the steep unbounded resonances that will result.
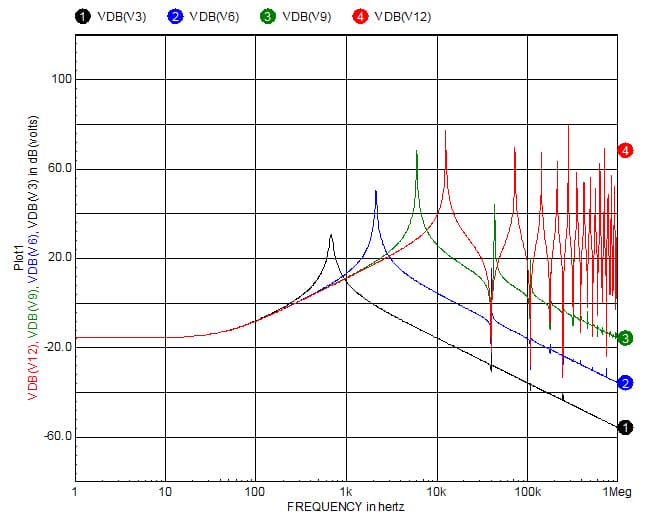
In Figure 3, the effect is given when PFC capacitors are placed at the load side, locally near to the end-user. In local impedance becomes fully overruled by the ideal PFC capacitors and all impedance resonances on the power cable in-between appear to vanish in the low impedance of the local power port observed. This doesn’t mean that the total mains distribution system is no longer suffering from resonances. Due to the local application of the PFC capacitors at the user’s end, steep resonances occur w.r.t. the noise currents injected into the mains distribution network, see Figure 4. Resonances continue to exist between the PFC capacitors and the transformer 1 km away, such that end-user generated noise is propagated all along the distribution network to the transformer and then further through the various mains distribution network branches connected to other users and severe crosstalk may result.
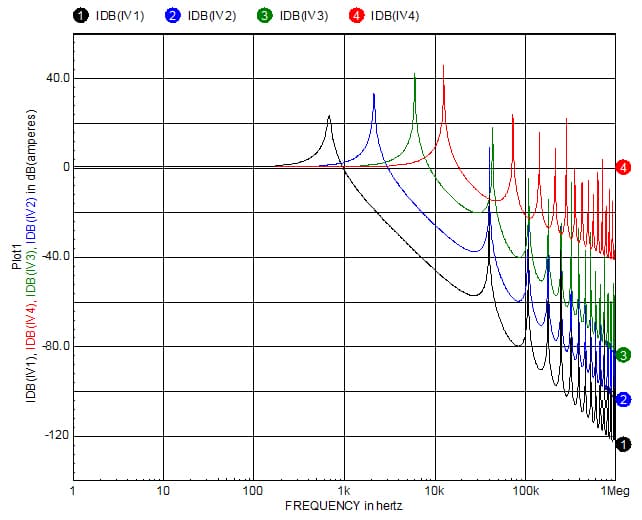
TUNING OR DE-TUNING
For the sake of optimizing the power efficiency utilization of the infra-structure at the operational mains frequency, the power factor shall be optimized (tuned) to reach unity. For the sake of avoiding resonances on the power distribution networks, RF-lossy filtering shall be applied to lower the quality factor at the steep resonance frequencies occurring. As such some power losses shall be introduced intentionally at the higher frequencies to control the impedances occurring on the mains distribution network in a better way. Moreover, the applied mains filtering shall be adjusted such that mains impedance resonances do NOT coincide with the operation frequencies of AIC, UPS, PWM and other switching regulators.
In a common domestic environment, we will have 50 or more switching devices being connected to the local mains distribution network. Not all at the same time and not all having the same impact on the noise voltages, currents or the mains distribution network impedance. Every charger, PC, laptop, TV, multi-media center will have its own switch-mode power supply (SMPS). Every solid-state driven LED or tubular lighting power controller is switching and all of them have built-in mains filters with X- and/or Y-capacitors at the mains side affecting the mains distribution network impedance locally. No port impedance requirements are given to those mains filters other that they have to ensure that the appliance filtered will be compliant when measured against a standardized artificial mains network (AMN) representing 50 Ω in parallel to 50 µH + 5 Ω, this for the frequency range 150 (or 9) kHz to 30 MHz. On the opposite side, no attention is given on what will happen if the ‘true’ mains impedances deviate too much from the standardized values or when multiple appliances are used in parallel.
For sure that all ‘50’ domestic reactive mains filters being interconnected to one another through the mains distribution network will result in a forest of resonances: 2(50-1) which will make power line communication near to impossible. Nevertheless, PLC modems (in the higher band) are offered with a data transfer of over 200 Mb/s with debatable performance.
When PLC is formally allowed and promoted with guaranteed performance levels, then the minimum of the effective mains distribution network impedances and the minimum of the ‘load’ impedances need to be better defined and controlled to enable conducted communication over the mains distribution network as a medium. Furthermore, a maximum channel/path loss shall be defined between several wall outlets in-between PLC is expected to be used, this under the given RF source and RF load impedances. An international standardization example is IEC 61334, Distribution automation using distribution line carrier systems – a standard for low-speed reliable power line communications by electricity meters, water meters and SCADA [1]. Most of the standards are PLC standards and protocols are industry driven like DC-BUS, HomePlug Powerline Alliance, HomePNA, IEEE 1901, IEEE 1675-2008, KNX is a standardized (EN 50090, ISO/IEC 14543), OSI-based network communications protocol for intelligent buildings), LonWorks, Multimedia over Coax Alliance, Residential gateway, Universal Powerline Association, etc.
Secondly, having a non-predictable mains impedance means that the noise generation and propagation along the mains distribution network is unpredictable unless isolation measures are being taken at the point of entry. The mains impedance proposal as outlined in IEC 61000-4-7 is not suited for standardization purposes. Having internationally agreed measurement impedances is a first step to quantify and qualify the generated noise by the systems connected. In the frequency range 2 – 150 kHz, a 1 or 10 Ω differential impedance is promoted in a draft IEC 61000-4-19 document. At the lower mains harmonic frequencies, standards exist: IEC 61000-3-2 for appliances up to 16 ampere/phase and IEC 61000-3-12 for appliances up to 75 ampere/phase where it needs to be emphasized that only at the power mains frequency the impedances are defined.
So far, no consensus has been reached on the need for regulation of interferences in this frequency band 2 – 150 kHz, being considered the ‘garbage’ band and considered a necessity to enable electronics to comply both at the lower frequencies: ≤ 40th harmonic and to the EMC requirements above 150 kHz. The economic impact is considered horrifying for industry on the necessary measures to be taken. No consensus has been reached about any regulation for PLC devices by CENELEC (SC 205) in the upper band. Erroneous readouts and responses are known from residual current breakers (RCBs) and electricity meters for which conducted immunity requirements are under development in IEC TC77A. No simple retrofit measures are known to overcome existing impedance resonances in mains power distribution networks and to limit the impedance effect of the reactive loads (at the higher frequencies) connected thereto by which coexistence between PLC and the mains power distribution can be ensured.
The actual problems of mains-borne noise below 150 kHz which are troubling the IEC’s EMC standards committees at this moment can be found in report SC205 here.
Different from the power mains frequency quality engineering approach that the mains voltage has to be monitored and that mains currents are just a result is only partly true as many of the mains distribution network impedance resonances are self-affected by all reactive components used: power factor correction as well as mains filtering, applied by the many devices connected and the cable length in-between.
CONCLUSION
At the international standardization level: IEC TC22, TC77A and at the European governmental level (CENELEC SC205) huge debates are going one at this moment as from the governmental side smart metering, using PLC, is promoted. Due to the unacceptable performances in the field of these smart metering devices, with PLC interfaces, this initiative is hampered. As an alternative, e.g. in the UK, Germany, smart meters will use wireless communications.
When smart-metering, with or without PLC as an interface, don’t come available on short notice, the self-generated energy: PV, wind, etc. cannot be fed back onto the mains distribution network economically reliable. Both parties on each side of the mains distribution network i.e. energy meter want to get paid for what they’ve expected to have delivered.
The use of PLC as a reliable communication path in either consumer or industrial areas is determined by a great number of parameters which might even be beyond the control of the end-user and will vary throughout the day.
Up until today, no international activities have been started by setting up requirements on mains impedance variability nor on the RF impedances a mains connected device may represent towards the power distribution network. Immunity requirements are under development: IEC 61000-4-16 and 19 (IEC TC77A) but based on circumstantial evidence as again mains distribution network impedances were left open during the interference voltage data gathering. As such, international standardization is lacking behind the facts, unable or unwilling to keep pace with technical developments.
REFERENCES
[1] EN 50065, Signalling on low-voltage electrical installations in the frequency range 3 kHz to 148,5 kHz. General requirements, frequency bands and electromagnetic disturbances, 2011, CENELEC, www.cenelec.eu
[2] EN 50160, Voltage characteristics of electricity supplied by public distribution networks, 2010, CENELEC, www.cenelec.eu
[3] EN 55011/ IEC CISPR 11, Industrial, scientific and medical (ISM) radio-frequency Equipment Electromagnetic disturbance characteristics. Limits and methods of measurement, 2007, CENELEC, www.cenelec.eu or 2009, webstore.iec.ch
[4] IEC 61000-2-5, Electromagnetic compatibility (EMC): Environment – Description and classification of electromagnetic environments, 2011, webstore.iec.ch
[5] IEC 61000-3-2, Electromagnetic compatibility (EMC): Limits – Limits for harmonic current emissions (equipment input current ≤ 16 A per phase), 2005, webstore.iec.ch
[6] IEC 61000-3-12, Electromagnetic compatibility (EMC): Limits – Limits for harmonic currents produced by equipment connected to public low-voltage systems with input current > 16 A and ≤ 75 A per phase, 2011, webstore.iec.ch
[7] IEC 61000-4-13, Electromagnetic compatibility (EMC): Testing and measurement techniques – Harmonics and interharmonics including mains signalling at a.c. power port, low frequency immunity tests, 2009, webstore.iec.ch
[8] IEC 61000-4-16, Electromagnetic compatibility (EMC): Testing and measurement techniques – Test for immunity to conducted, common mode disturbances in the frequency range 0 Hz to 150 kHz, 2011, webstore.iec.ch
[9] IEC 61000-4-17, Electromagnetic compatibility (EMC): Testing and measurement techniques – Ripple on d.c. input power port immunity test, 2009, webstore.iec.ch
[10] IEC 61000-4-19, Electromagnetic Compatibility (EMC): Testing and measurement techniques – Test for immunity to conducted, differential mode disturbances in the frequency range from 2 kHz to 150 kHz, at a.c. ports, 2012, committee draft, webstore.iec.ch
[11] IEC 61800-3, Adjustable speed electrical power drive systems – Part 3: EMC product standard including specific test methods, 2004, webstore.iec.ch
[12] IEC 61800-5-1, Adjustable speed electrical power drive systems – Part 5-1: Safety requirements -electrical, thermal and energy, 2007, webstore.iec.ch
[13] IEC 62040-1, Uninterruptible power systems (UPS) – Part 1: General and safety requirements for UPS, 2008, webstore.iec.ch
[15] IEC 62040-2, Uninterruptible power systems (UPS) – Part 2: Electromagnetic compatibility (EMC) requirements, 2005, webstore.iec.ch
[16] IEC 62103, Electronic equipment for use in power installations, 2003, webstore.iec.ch
[17] http://www.iec.ch/about/brochures/pdf/technology/transmission.pdf