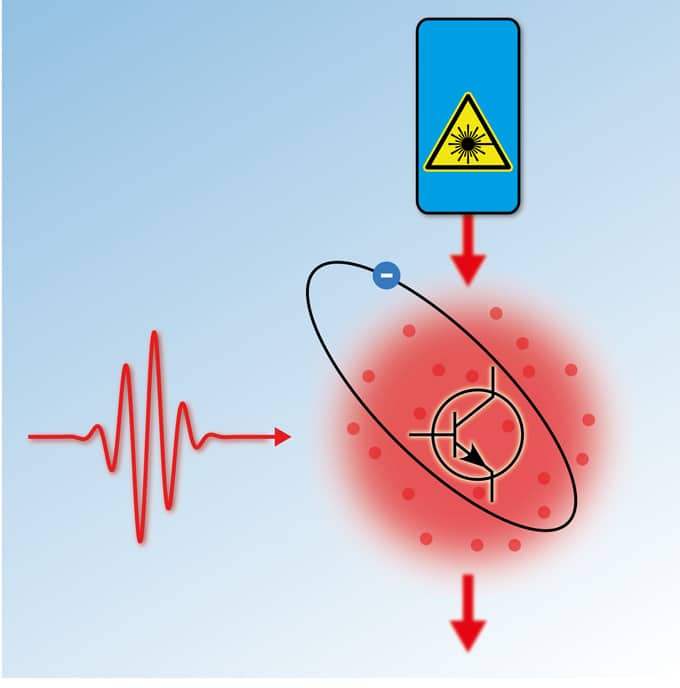
Using an ultracold quantum gas, researchers at the Max Planck Institute of Quantum Optics in Germany have created a new kind of transistor-like optical amplifier that allows for a twentyfold amplification of single-photon signals.
Although fiberoptic technology has helped speed along data transmission and telecommunications in general, researchers have hoped to find another method of data processing that uses only optical techniques (i.e. optical transistors and optical logic gates), and not on glass. Such a method would dramatically improve the performance of quantum processing, which relies on information being stored in short optical bursts that contain only a single photon each.
In research published in Physical Review Letters, scientists in Germany have created a new kind of optical transistor that uses a cloud of ultracold rubidium atom to amplify single-photon signals up to twenty times their usual strength, potentially leading to a future of ultra-fast all-optical data transmission.
In transistors, a large amount of gain – the effect that a change in the input signal has on the output signal – is needed to distribute the output signal to the input ports of multiple transistors without weakening the signal, becoming a necessity for building complex digital switching circuits.
According to an explanation found in a recent MPQ press release, in optical transistors, the input signal – a weak light pulse known as a “gate pulse” – is used to modify the transparency of a chosen medium for a second “target” pulse. This phenomenon is known as “electromagnetically induced transparency” (EIT), and makes the medium transparent for light pulses of certain frequencies.
The chosen medium is irradiated with two lights pulses of the same color, the second occurring two microseconds after the first. The first pulse, or “gate pulse,” contains less than one photon on average, and is very weak. Applied alongside the applied control laser, the gate pulse brings one rubidium atom in the cloud into a highly-excited Rydberg state, causing one of its outer electrons to orbit far away from its nucleus. However, the press release explains, rather than being contained to itself, the Rydberg atom causes the other atoms in the cloud to shift energy levels. By the time the second “target” pulse hits the cloud, the cloud’s colors does not match its EIT conditions any longer. Because of this, the target pulse is blocked by the atoms.
The chosen medium for the MPQ experiment was a cloud of roughly 150,000 rubidium atoms contained within an optical dipole trap, generated by two laser beams. The cloud was kept at a frigid temperature of just above absolute zero, at about 0.30 micro-Kelvin, and could be held in place for several seconds at a time.
MPQ researchers demonstrated earlier this year that it is possible to modify the properties of rubidium atoms with single photons. However, this previous experiment operated under severely limiting constraints, including the duration and intensity of pulses used, that the current research project successfully avoided.
“In the present experiment we have changed a few things, most importantly, we use control lasers with different wavelengths for gate and target pulses,” lead scientist Stephan Dürr, Ph.D., explained in the press release. “That way we avoid that the target pulse couples to the Rydberg excitation and retrieves the gate pulse, even for long durations of the target pulse.”
Additionally, the researchers manipulated the Rydberg states to achieve a Förster resonance that caused the Rydberg atoms to interact with each other more enthusiastically than usual.
“The Förster-resonance enhances the effect of the Rydberg blockade which is the true mechanism that prevents the target pulse from traversing the atomic cloud,” Ph.D. candidate Daniel Tiarks, also working on the project, said. “Furthermore, with the principle quantum numbers of the chosen Rydberg states we get a smaller effect of self-blockade of the photons in the target pulse, another obstruction that we had to deal with in our previous experiment. With all these measures we were able to increase the duration of the target pulse by two orders of magnitude, up to 200 microseconds.”
The researchers compared the intensities of the outgoing target pulses with and without a prior single-photon gate pulse, and found that the target signal was successfully reduced.
“Right at the Förster-resonance we observe a reduction of 20 photons,” Dürr said. “This effect should make it possible – at least in principle – to cascade such transistors in order to solve complex computational tasks. In addition, the present experiment demonstrates a new and non-destructive method for the detection of Rydberg excitations. Because of the high amplification we can reveal whether a single Rydberg excitation has been created in the atomic cloud in a single shot.”
With its impressive gain, this new optical transistor could allow scientists to distribute a transistor output to the inputs of multiple transistors, enabling the achievement of complex computational tasks.
– Melanie Abeygunawardana