Don White
Consultant, Don White Consultants
Jerry Emanuelson
Consultant
Part 1 of this article addressed a proposed national plan for U.S. EMP protection. Part 2 of the article presents methods and techniques for EMP protection of buildings, solar rooftops and other structures. As such, Part 2 covers details of shielding, bonding, grounding, and cable or device surge suppression and filtering. These apply to structures from sheds and rooms to small and large homes, and to commercial and industrial buildings less than about five floors in height.
SHIELDING A HOME & BUILDING BEFORE A SOLAR ROOFTOP
Electromagnetic shielding works from a combination of (1) radiated emission reflection loss at the metal shield and (2) metal absorption loss. The first component develops from the ratio of the arriving wave impedance and that of the metal. The second comes into play when the skin depth of the metal exceeds about 50% of the metal thickness.
Shielded Building, Rooms & Cabinets

cabinet or box.
Figure 8 illustrates that there exists three levels (possibly more) of shielding. The benefit of shielding the entire building to 80 dB (amount discussed earlier) means that nothing else inside needs to be shielded. So existing climate controls, cell phones, iPhones, iPads, laptop computers, peripherals, and all the rest of electrical and electronic devices can continue to be used. However, shielding a building to 80 dB in some cases become expensive (for example, more than 10% of the entire building cost).
Consequently, one or more internal shielded rooms could be added where the more sensitive items would be located, and the entire building shielding can be correspondingly reduced. For example, suppose aluminum foil or sprayed copper paint on the outside walls, ceiling and floor (bonded and secured) are used to produce 45 dB shielding. This reduces the needs of the remaining shielded rooms, cabinets or enclosures to 35 dB as shown in Figure 8.
However, if the same skin shielding were applied to the inside of the outer walls, this would not work as the electrical wiring in the outside walls is still not protected.. Thus, apply the shield to the outer building skin .
If the internal building items have a certified radiated susceptibility limit of 10 V/m (the assumed typical conditions), the remaining shielding required can be achieved in an internal screen room or cabinet or box size, as applicable. Shielded rooms of the quality offered by the EMC manufacturers are shielded to about 120 dB and represent a huge overkill in performance and price, and therefore, would not be used here.
The applied shielded building skin is therefore placed on the outside with sheet metal building walls bonded where joined. Each of the six sides of the building (roof, basement floor, and four sides) is bonded along its entire length.
Figure 8 shows that the windows, rooftop elevator shack, A/C heat exchanger, and all cable shields penetrating the building must not have SE less than 80dB below 64 MHz. (See Figure 17.)
Shielding of the Building Facade
How to shield the building facade (outer skin)? What constitutes the basic material under the building skin or envelope, such as with vinyl siding? In the U.S., it is typically a plywood lath sheathing in the North and concrete blocks (stuccoed over) for residential in the South. For commercial it may be aluminum composite panels, copper or stainless steel sheets, weather-board, etc. These and other materials are dependent on whether or not the building already exists or has not yet been designed or built. Most specifics of this discussion are beyond the scope here and involve important architectural matters.
If the facade of the existing building permits the direct addition of an aluminum foil, then the household, 1 mil (= 0.001 inches = 0.0254 mm) or a more ruggedized version (for example, 3 mils) of aluminum foil provides all the shielding needed.
One mil of aluminum foil provides 96 dB of shielding. So there exists sufficient shielding. But how are sheets of foil to be bonded to their mounting material siding? Also, how are they mated together at their edges?

Basically, an adhesive spray is made on the mounting material back and the foil is placed thereon. However, the foil overlap should approximate one inch (2.5 cm), as shown in Figure 9, and a masking tape used to secure the overlap junction. Do not spray the foil adhesive in the 2.5 cm region as metal must be bonded to metal without any other material in between to ensure a high conductivity and good shielding.
The foil must end at each window sill or outside door sill or frame as the window/door will receive its own shielding. An electrical bonding agent or gasket (described below) is used to electrically connect the building facade with each window and door periphery.
One possible option to the above foil is to use copper or aluminum paint, applied by brush, roller or spray. A finishing non-conductive protection coat of latex paint is applied. One major benefit of the paint approach is the relatively easy application by spraying and the avoidance of foil overlaps, and electrical gaskets except at windows, doors, etc. described below.
How to close this gap? There are different methods, but they go beyond the level of this article. Of course, to a new building just being planned or being built, this is not an issue as the bottom floor shield is extended beyond outer frame footprint to be bonded to outside shielding.
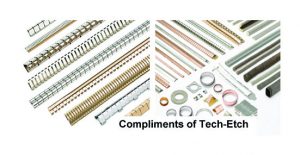
Regarding electrical gaskets used to seal the adjoining metal sides, Figure 10 shows options available from companies like Tech-Etch for the engineering community. The figure also shows other electrical gaskets use in bonding other types of mating electrical parts.

Grounding of Shielded Building
Figure 11 shows the shielded building including building skin, shielded windows, shielded HVAC and elevator shacks and other external elements. The shielded building could float in space without grounding, and the shield works fine. No grounding is required. However, there are other concerns such as if the shield becomes charged up to any voltage above ground level due to whatever stray radiations (or accidental connection of a hot AC lead to building skin), it constitutes a shock hazard to entering and exiting people. Also, lightning control must be considered. That’s why the building skin must be grounded (earthed) to satisfy the National Electrical Code.
Also, as shown at the lower left, Figure 11, telecom leads, control and signal leads, and AC power leads must enter the building. These cables must also be shielded. Since the cable shield and the building shield may be at a different voltage, the entering cable shield must be bonded to the building shield (usually a metal plate. The object is to divert the EMI (induced electromagnetic interference shield currents) and grounded to earth, where they are harmlessly dissipated. But the devil is in the details.
The sketch of Figure 11 illustrates the situation repeated thousands of times throughout a state or a country of a developed nation. Radiated EMI (or EMP) is coupled to a cable shield where EMI surface, common-mode currents, Ic, are grounded at the shielded building entrance (assuming a best case in which the building is shielded). It is typically assumed that the EMI currents will flow down the ground rod(s) (see Ig) and dissipate harmlessly into the absorbing earth. Some EMI currents (Is), however, still flow on the building shield (that skin current is capacitive coupled back to ground to close the loop). Those currents may then penetrate into the building skin where they can upset or burnout internal victims. So, how successful (e.g., how low?) is the low-impedance quality of each? Also, how much attention has been paid to pertinent details?
Remember, the only shield current that should flow is from the terminated, direct radiated EMI onto the building shield, per se. It should not contain additional currents that come from any cable entry ground which can exceed the terminated EMI surface current on the building by large amounts. The degree to which it is exceeded is a direct measure of the reduction loss in building shielding effectiveness.
If the building were built on sand or some non-moisture holding soil, or a drought existed in the area, then the grounding system will not work as well (or poorly). If the ground Fargo clamps eroded out or became disconnected, then what? (Note: Measure-ment of Soil Resistivity, in MIL-HDBK-419 speaks in detail to the number of ground rods, ground wells and earth electrode subsystem necessary to achieve the grounding objectives).
Can you testify if your own facilities or other installations are properly grounded? What criteria or standard do you use? Who signed off on the inspection? It is still “grounded” five years later?
As it develops, the entire discussion may be academic from an EMP point-of-view since few non-government buildings are EMP protected (shielded) in the first place today. For those situations, all the interior electronics are fried in an EMP event – grounded or not.
EMP, lightning and EMI won’t go away. Grounding is an important part of the protection system, but shielding and filtering are of equal concern.
Shielding Windows, Doors & Other Building Skin Leakage

Windows cannot employ electro-chrom glass as this blocks light with little shielding, nor can one have a few microns (very thin film) of vapor-deposited metal on the window as a shield since one could not then see through the window. Although a one micron (very thin film) of silver deposited on glass offers an RF shielding effectiveness of 80 dB, it also blocks the optical transmission.
Therefore, a metal screen mesh (Figure 12) is considered for covering the windows which passes almost all the light (optically) but blocks the EMP radiated transmissions by 80 dB below 12 MHz (depends on the mesh screen spacings). The required screen mesh element separation distance, d, to achieve this shielding, is calculated by:
SEdB=20Log10(150/(d*fMHz) = 80 dB
where: fMHz = 12 MHz from the geometric mean of the first and second corner frequencies in the Bode Fourier frequency plot corresponding to the 5 nsec rise time of the standard 150 nsec EMP pulse.
Solving the equation for mesh separation, d, produces a distance of d = 1.29 mm. This corresponds to 25.4/1.29 or 20 wire mesh openings per inch (OPI). This OPI is mentioned since mesh shielding is usually reported in OPI by manufacturers, and is available up to 100 OPI.
For shielding solar panels, do not use higher OPI as the light transmissivity begins to suffer and this will adversely affect the solar panel efficiency of converting sunlight into electricity.
Shielded External Doors
One or more doors permit entry into and exit from the shielded building. For each such door, a vestibule must be generated such that a set of two doors is used with interlocks so that not more than one door can be opened at any time. This will avoid a fortuitous situation in which both doors are temporarily open at the moment an EMP incident occurs, which would otherwise compromise the entire integrity of the building shielding effectiveness.
Shielded Wires and Cable Entries
As shown in Figure 11 earlier, three cable and wire classes enter nearly every building: (1) telecom (telephone, Ethernet, and other hard-wire communications) leads, (2) control and signal leads for controlling certain activities and reporting back elsewhere with status data, and (3) AC power mains to supply 120 VAC, 240VAC or other voltage to run the building’s many operational loads previously discussed. Unless the cables are already placed in conduit, buried and/or shielded, their shields must provide the required 80 dB shielding below 64 MHz isolation to the entering leads.
The interconnecting wiring between and among all solar panels, inverter (if micro-inverters are not used) and the down conductors to the service entrance panels must also be shielded to 80 dB. A solid thin wall copper tubing can also be used as the shield. However, to ensure the benefits of flexibility, knitted-wire mesh shielding can be used on all cabling instead. All shielding must have an overcoat of weather sealant or outer jacket if not placed inside a conduit.
As explained above, the cable shields are bonded to the building shield usually at a metal grounding plate welded to the building shield at cable entrance (see above discussion on building grounding).

Adding a Shielded Solar Rooftop
An EMP unprotected solar rooftop typically consists of panel mounting racks, solar panels, interconnecting cables, one inverter (or a micro-inverter at each panel), down conductor cables to a power-select transfer switch (connects utility power or solar panels), and backup batteries. Batteries are used for nighttime power and overcast days when the solar panel power output is insufficient. Generators are not normally used since unless protected they would burnout in an EMP burst, and they require fuel which may not be available after an EMP incident.
Solar panels usually contain 1st generation silicon solar cells (the work horse for generation of space electricity for 40 years) which have the highest light conversion efficiency. The panel frame usually measures about 3 feet (0.91 meters) x 5 feet (1.52 meters) and produces an output of about 200 watts with a 22±% light-conversion efficiency. Increasing in popularity is CaTu, a second generation, thin-film, solar cells costing significantly less (First Solar of Tempe, AZ has produced these at a reported production cost as low as $0.52/watt), but they have a conversion efficiency of about 65% of silicon.
The average home size in the U.S. is 2,300 sq. ft (214 sq. meters). A one floor house will have a roof of about 2,700 sq ft (252 sq. m). If about half is facing roughly south and 80% is useful to hold the solar panels, then up to 72 solar panels can be mounted.
Because the solar panels are not pointing at the sun (i.e., fix mounted with no tracker, such as the trackers used in solar farms) during the rotation of the earth, the average power output over a 10-hour period may be about 10 kW. If the home has two floors, then available roof provides about half this or about 5 kW. By adding a typical garage, this may add another kW. The average house in the U.S. requires about 8 kW to handle the A/C, hot water, clothes washer/drier, appliances, lighting and other loads.
The average of 5 kW applies for about five hours per day and falls off as the sun moves toward the horizons. In the northern hemisphere, there is less in winter and higher latitudes and more in summer and lower latitudes. The above assumes no blockage due to the shadows of other buildings and trees.
Of course, a solar installation would be instantly burned out in an EMP incident, so the entire installation must be EMP protected.
Adding Shielding to Solar Panels
After mounting the framework racks on the building roof, the shielded solar panels are then affixed thereto. The shielding is to provide the required nominal 80 dB solar panel shielding:
Shield the panels completely inside of the solar glass window face and bond them to the frame which provides complete metallic coverage of the other five sides. The solar screen may be 20 OPI (openings per inch). welded screen mesh, previously mentioned. This shielding is to be done back at the factory where the panels are manufactured for quality control and long-life performance. The screen will reduce the light transparency by roughly 15%.
Adding Shielded Micro-inverters or In-verter (shield only)
The micro-inverter is a small DC-AC converter which takes a typical 12 VDC output from a solar panel and converts it to 120 VAC or other user load value. As appropriate, the panels are connected in series and/or parallel to produce the desired load voltage. A small micro-converter can be built into the shielded solar panel near its output connector (this is to be done at the time of solar panel assembly back at the factory) or mounted separately next to the panel. Unless the entire solar rooftop assembly is to be shielded separately, the shielded unit requires an HV surge suppressor at the cable connectors.
Micro-inverters have several advantages over conventional central inverters. Even small amounts of shading debris or snow lines on any one solar panel, or a panel failure, does not disproportionately reduce the output of an entire array. Each micro-inverter obtains optimum power by performing maximum power point tracking for its connected panel.

Their primary disadvantages are that they have a higher equipment initial cost per peak watt than the equivalent power in a central inverter, and micro-inverters are normally located near the solar panel, where they may be harder to maintain. These issues are usually surpassed by micro-inverters having higher durability and simplicity of initial installation.

When the total rooftop solar load exceeds about 10 kW, micro-inverters are usually not used and the inverter takes over. The photo in Figure 14 shows three inverter sizes ranging from 10 kW to 500 kW. If they were located outside the EMP shielded building they would have to have cabinet shielding of 80 dB plus cable surge suppressors and shielded cabling. To avoid this, if possible, they should be placed inside the 80-dB shielded building (e.g: garage) and existing models used with no further shielding retrofit required.
Adding EMP Surge Suppressors and Filters
Commercial electronic surge suppressors used for lightning control do not clamp fast enough (clamp >100 nsec for lightning); need to clamp less than 5 nanoseconds for EMP, to protect against the near instantaneous effects of an EMP. Some also may not have great enough current carrying capacity. So it is important that already existent EMP surge sup-pressors be used for any exterior wire/cable entrance from the outside world to the inside of the EMP-shielded building.
Figure 15 shows two of several HV EMP surge suppressors available for addressing the wide variety of needs and applications. (The surge suppressors that are sometimes used for high voltage transformers in substations to address the geomagnetic storm needs are altogether different and are not shown here)
Adding the Shielded Smart Meter

A smart meter (Figure 16) is usually an electrical meter that records consumption of electric energy in intervals of an hour or less and communicates that information at least daily back to the utility for monitoring and billing purposes.
Smart meters enable two-way communications between the meter and the central system. Unlike home energy monitors, smart meters can gather data for remote reporting. Such an advanced metering infrastructure (AMI) differs from traditional automatic meter reading (AMR) in that it enables two-way communications with the meter. Some are also equipped to do much more.
Since the smart meter is mounted on the outside of a building, it will have to be shielded to 80 dB to match the EMP threat. This can be accomplished by using the same 20 OPI wire mesh screen engulfing the entire meter on the front and back as discussed earlier for windows and panels.
Note that smart meters are more EMI susceptible than their replaced older meters. So the smart grid is more susceptible if it is using on-line digital generation and computation of data.
EMP TEST AND CERTIFICATION
Testing the Solar Installation
Contractually, it is necessary in performance tests to demonstrate EMP-protection compliance with the 80-dB shielding requirements over the designated frequency spectrum.
While the building EMP and solar EMP compliance tests can be done simultaneously, in the early stages it may be best to do each separately. This will facilitate diagnostics-and-fix as needed. The building compliance test must be done first. Details are beyond the level of this article and will not be discussed here.

EMP Test and Certification
How does one know if the installed EMP pro-tection will work (cause no electronic failures) if or when an EMP burst happens? Was an installation over or under protected and by how much? Of course, without an insitu testing, it may never be known. One does not want to pay for something if it cannot be demonstrated to work.

Instead of a simulated EMP burst, seven or more test frequencies are used in accordance with the Bode Plot shown in Figure 17. Otherwise, testing would be impossible since all other devices in the area would be burned out.
As mentioned in MIL-STD-188-125, the EMP pulse in the time domain (X-axis is time) has an amplitude of 50 kV/m, a rise time of 5 nsec and a pulse width at the half amplitude of 150 nsec. This is shown in the insert at the left within Figure 17 and represents the test specifications for EMP testing and compliance.
Figure 17 also depicts the frequency-domain (X-axis is frequency) manifestation of the time-domain pulse just mentioned. It is presumed here that all electrical and electronic devices comply with European Union (CE mark) or other test compliance of radiation susceptibility limits of 10 V/m. (For example, the European Union specifies in EN61000-4-3 that equipment subject to Level 3 requirements shall not be susceptible to a field strength 10 V/m over the frequency range 80 MHz to 1 GHz.
While testing details are beyond the scope of this article, in concept testing is quite simple. A small van or pickup truck at the left in Figure 18 contains a scanning oscillator or sweeper which feeds a power amplifier which drives radiating test antennas pointing at the building to the right. Inside the building is a tracking receiver driven by matching pickup antennas.
The test configuration is first calibrated as a reference with both transmitter and receiver configurations on the outside (step 1). Tests are made at the seven frequencies indicated in the drawing, and the RF attenuator settings recorded. Then, maintaining the same distance between transmitter and receiver, both are moved as shown in step 2 with the receiver configuration moved mostly to the middle of the building and attenuator settings again recorded to get the same levels as in step 1. The difference between the attenuator settings in step 1 and step 2 constitutes the building shielding in dB.
The tests are repeated for the other remaining three (or more) sides of the building, recording the shielding effectiveness of each side for all seven frequencies. Then for each frequency, the corresponding four side shielding effectiveness are compared and the smallest dB value selected. These results constitute the building EMP shielding effectiveness. They are compared with the Figure 15 Bode plot to determine compliance.
There is a more automated procedure than just explained. It is discussed elsewhere. Also, if the building is large (several floors or acres in size), the transmitter may be located in a helicopter or blimp.
Maintenance Considerations
Everything ages, including all buildings and their contents. For the outside, the more severe the climate and weather, the more rapid degradation and variance in performance is expected. In addition to shock safety, building grounding will determine how well the building EMP shielding has held up. As stated earlier, the soil loaminess (somewhat dark, and good for growing grass or other vegetation) makes for good grounding as it holds moisture. Arid soil like sand is very poor and low on conductivity. It may need to be impregnated with bauxite (an aluminum base particulate) or proprietary grounding compounds to permit better earthing into a much lower impedance sink.
As observed, testing for grounding effectiveness will show up in the above open air shielding effectiveness tests. So a building’s earth and grounding should be tested by a licensed and certificated technician to add to the insurance protection in some periodic basis. Initially, use every two years as a default period.
SUMMARY
This article, A National Plan for EMP Protection, has been presented in two parts. Part 1 addressed a plan to EMP protect communities, villages, towns and small cities in sufficient quantities and sizes to maintain some defined semblance of our civilization lifestyle following an EMP burst. This is to be contrasted to an EMP survivalist who survives but surrenders his lifestyle.
The second part, Part 2, addresses the shielding of small buildings up to large houses, small and large commercial shopping malls and industrial manufacturing and big box replenishment structures. Details including shielding, bonding, grounding, surge suppression and filtering were covered. These actions generate new products and services, new
The second part, Part 2, addresses the shielding of small buildings up to large houses, small and large commercial shopping malls and industrial manufacturing and big box replenishment structures. Details including shielding, bonding, grounding, surge suppression and filtering were covered. These actions generate new products and services, new markets and create millions of new jobs.
ABOUT THE AUTHORS
Don White, registered professional engineer, retd., holds BSEE and MSEE degrees from the University of Maryland. He is past CEO of three Electro-magnetic Compatibility companies in metro Washington, D.C.
Don has written and published 14 technical books over a span of 30 years. His last book was The EMC, Telecom and Computer Encyclopedia, an 800-page compendium.
At Don White Consultants, he published a bimonthly trade journal called EMC Technology magazine circulated over four continents. He was the technical editor and wrote many of the tutorial articles. Don is past president of IEEE Electromagnetic Compatibility Society. He may be reached at 941-743-8100 or DonWhite@emp-safeguard.com or drjw9@aol.com.
Jerry Emanuelson holds a BSEE degree from the University of Colorado. He began his career as an audio and video test engineer for Ampex Corp., the manufacturer of audio and video tape recorders. He later became the Transmitter Supervisor for a broadcasting company with transmitter locations on mountaintops, subject to severe lightning and significant EMI problems due to many types of closely packed transmitters.
Jerry is a leading EMP Internet author with scores of EMP-related subjects. He is a former member of IEEE Broadcast Society, and is now part-time electronics consultant and a part-time non-fiction science writer. Jerry can be reached at emp@futurescience.com