The demand for wireless medical applications such as remote patient monitoring, real-time diagnostic analysis, smart surgical systems, and implantable sensors have grown significantly over the last couple of years. Thanks to implanted medical devices, many patients live an almost normal life without having their mobility compromised. Popular implants include cardiac pacemakers, implantable defibrillators, nerve stimulators (functional electrical stimulation, FES), bladder stimulators, implantable infusion pumps, biomonitoring devices such as the capsule endoscope and implantable drug delivery systems. A patient’s quality of life is vastly improved by implants using wireless communication; the risk of inflammation and infection, often caused by connecting wires and tubes, is greatly reduced, as well as being much more convenient.
The wireless medical device segment that has seen perhaps the biggest jump in demand in recent years is remote patient monitoring. One of the biggest driver of the soaring demand is a perhaps the pandemic and the due to its nature, healthcare is invariably understaffed with the largest shortages in trained nurses and doctors. Wireless remote patient monitors (WRPM) can augment conventional electrical monitoring devices to help reduce direct contact with individual patients while a doctor can still monitor the vital conditions at all times. WRPM also enable a doctor to monitor multiple patients at the same time remotely, thus freeing up valuable time to attend to the needs of the patients who need immediate attention. Not to mention, WRPM give the patient far greater freedom of mobility by removing the danger of getting entangled with wires. Moving patients between locations while continuing monitoring is also a great deal easier.
How do remote patient monitors transmit data?
Typically, a wireless medical device utilizes both the 400 MHz MedRadio band and the 2.4 GHz MBAN band. The sensor data and control data is sent back and forth on different frequency bands. Increasingly, state-of-the-art patient medical devices are taking advantage of commercial standardized technologies such as WLAN, Bluetooth®, Zigbee operating in the unlicensed ISM band. In certain applications, depending on the data rate requirements, where mobility is required, LTE or LPWAN (LORA & Sigfox) based implementations are also gaining popularity.
A WRPM gathers the patient’s vital signs data (such as body temperature, pulse rate, respiration rate and blood pressure) from wired measurement sensors and then wirelessly transfers the data via the Wi-Fi access point to the health care facility’s server system for storage at a central location. Medical personnel can then tap into the patient health status data by accessing it from the relevant server location using a networked connection. Figure 1 shows the intended WRPM architecture in a hospital environment.
Future versions of WRPM systems will also support Bluetooth®-based vital condition data gathering, which means that the sensors will communicate with the WRPM wirelessly and allow the patient with even more mobility and comfort.
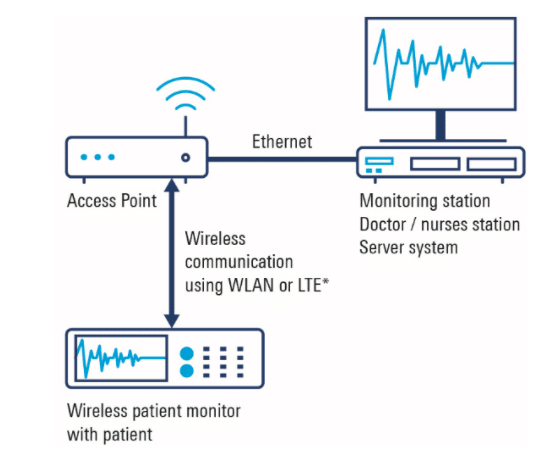
RF spectrum and interference
A WRPM is mostly used in a hospital. Nevertheless, there is a broad range of potential use cases that includes elderly care homes, military & defense applications and in some cases even at a patient’s home. This means that the devices are subjected to very different environments for Radio Frequency (RF) transmissions. In many day-to-day environments, there is an abundance of wireless electronic gadgets, most of which also operate using licensed and un-licensed technologies such as Bluetooth®, WLAN, cellular mobile radio, etc. Depending on the density of connected devices, the RF environment gets very noisy, particularly since the most data-hungry radio products operate in the ISM unlicensed bands; normally using the 2.4 GHz frequency band.
WRPM is exposed to a variety of RF environments depending not just on the application. If we consider just the hospital application use case, depending on the location of the hospital, the RF environment differs quite a bit as well. A hospital in a big city usually sees more patients, and has more staff members and visitors than one in a rural location. In the big hospital in a city, more people bring in more mobile phones, smart watches, wireless car key fobs, Bluetooth® headphones, and so on. All these devices transmit signals in the same frequency band at which the WRPM also operates. The microwave in the staff kitchen also radiates high levels of RF energy at 2.4 GHz. In addition, there is bound to be a high-powered LTE Base station tower nearby that could also act as an interference source for the WRPM and further complicate the coexistence challenge. Most hospital IT systems prefer to run regular system updates wirelessly in the night since the RF spectrum is relatively “less busy”. Nevertheless, this also acts as an interference source for the WRPM.
What happens if there is a source interfering the communication of the WRPM?
The sources of the interference signals are smartphones, smart watches, smart home appliances, IoT devices(smart tooth brush, smart lights etc), WLAN routers, car doors openers etc, which are running cellular and non-cellular services such as Bluetooth®, WiFi hotspot services etc. Not to forget, medical devices may be also be subjected to intentional jamming attacks.
When there is an interference source operating at the exact same frequency (i.e. overlapping RF interference signals) as that of the WRPM, the data transfer between the WRPM and the server will become slower (i.e the data rate of the transmission will drop). In the extreme worst case, the communication will completely break down and absolutely no data will be transferred. If the interference signal is on an adjacent frequency channel than depending on the power level of the blocker, the data rate will also be lower. A drop in data rate means the monitoring stations does not receive the patient health information instantly but with an added time delay. In the worst case, medical personnel are not informed about life-threatening changes in the vital conditions at all.
What can be done to protect WRPM from interference signals?
Even though LTE is designed with interference mitigation algorithms to identify and avoid blockers, WLAN receivers mostly suffer in the presence of one or multiple blockers. Therefore, the best approach is to design robust receivers with good filtering capabilities. In order to ensure receiver robustness for wireless medical devices in the US, the FDA recommends product compliance according to the ANSI C63.27 and AAMI TIR69 standards to ensure minimum performance.
However, technology evolves much faster than standards. It is therefore important in the product development phase to select test methods that future-proof a product for its lifetime. A failing product even a few years after market placement can still hurt the brand reputation and can be subjected to heavy fines and penalties.
Regulatory requirements for wireless remote patient monitoring devices
The ANSI C63.27 specification is the only standard focused towards wireless coexistence testing. It is a mandatory requirement in the US, relevant devices must be complaint according to the guidelines provided in the standard. Canada, the EU and APAC countries do not have any special requirements for medical devices to be specifically wireless coexistence compliant. By the end of 2020, there will be 20 Billion wireless connected products. This means that, with the growing number of wireless products crowding the RF spectrum, hospital authorities have more confidence in products that can demonstrate interference robustness due to compliance to a certified and recognized standard (i.e. the ANSI C63.27 guidelines). All hospital administrators are interested in conformance to guidelines as a way of reducing legal liability in case of product failure that can be traced back to a wireless coexistence issue.
Thorough testing will save lives
The ANSI C63.27 standard describes wireless coexistence test using four different types of test setup. The most reproducible and realistic way of testing any wireless receiver is by performing radiated over-the-air (OTA) testing inside fully anechoic chambers.
A test plan needs to be generated that takes into consideration the risk assessment analysis for the product based on the intended use case. A product that is categorized as high risk (i.e. in the case of malfunctioning due to a coexistence-related issue, resulting in bodily harm to the patient) needs to be tested using a more sophisticated interference strategy that better simulates real world worst-case conditions. The risk assessment should take into account the wireless technology supported by the device, including the frequency and the exact bands supported as well as channels available on the radio module. The worst-case operating condition needs to be defined for the product itself and a method of evaluating the functional wireless performance (FWP) in both ideal and worst-case operating environment. The risk assessment outcome dictates how stringent the test for compliance needs to be.
The T&M challenges include, firstly, recreating the electro-magnetic environment applicable for the product’s intended use while performing coexistence tests to monitor the defined functional wireless performance (FWP) in a controlled measurement area. Secondly, receiver robustness and application level testing for intentional and unintentional frequency jamming using realistic interference signals in a repeatable manner.
Test & measurement solution
The ideal solution includes a radio communication tester, which can emulate all the wireless network technologies present in the typical working environment for the device (such as 3G, 4G, 5G, Bluetooth®, WLAN, ZigBee). Such a radio communication tester is an extremely powerful instrument since it can be used to replicate the hospital network, and it gives the user full control over the RF parameter configuration of the intended network. Additionally, it also includes the ability to monitor various functional wireless performance parameters such as data throughput, PER, BLER, as well as track IP packet data flowing through the network. Additionally, it is possible to measure IP security with this device. Figure 2 shows how a radio communication tester can simulate a commercial access point and replicate a real world network in a controlled testing environment. The baseline FWP is determined in ideal conditions without any interference signals present using this setup.
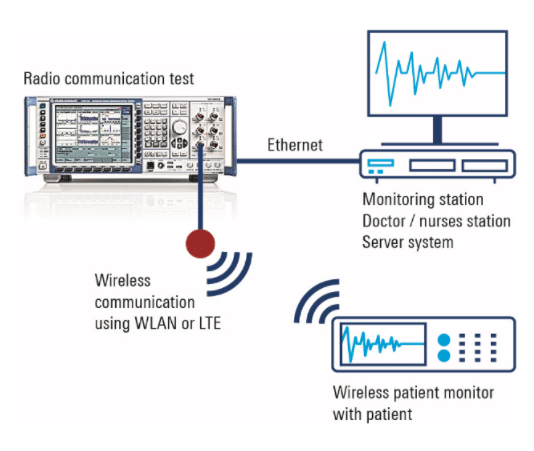
Depending on the risk categorization of the medical device being tested, the number of interference signals need to be adjusted. For ANSI C63.27 compliance testing, up to three interference signal sources are recommended. However, given the vast number of technologies and the quantity of interference sources (smartphones, smart watches, etc.) around us, it is recommended for R&D labs to test using an even higher number of interference sources, in order to fully characterize the WRPM device performance when the receiver is in a “fully stressed” RF operating environment. Figure 3 shows a test setup with an interference station with up to eight fully calibrated interference sources. Vector signal generators are used for generating interference signals, and should be capable of generating wideband-modulated signals, and able to flexibly adjust to the center frequency and the output power level of the unintended signals.
While performing coexistence testing, it is important to monitor the RF spectrum, as is listed as a mandatory step in the standard. A swept-tuned spectrum analyzer is adequate, but in some cases a real-time spectrum analyzer may also be required if very fast digital signals need to be captured. Almost all WRPM come with a display screen and a built-in loudspeaker system as an integral part of the functionality. An inspection software can be used to detect and monitor video and audio related parameters. The inspection software automates the detection process and also removes human errors completely from the display monitoring. Figure 3 shows the R&S®AdVISE inspection software, a powerful T&M tool that uses any USB based HD webcam and microphone to collect live data in order to monitor audio and video performance.
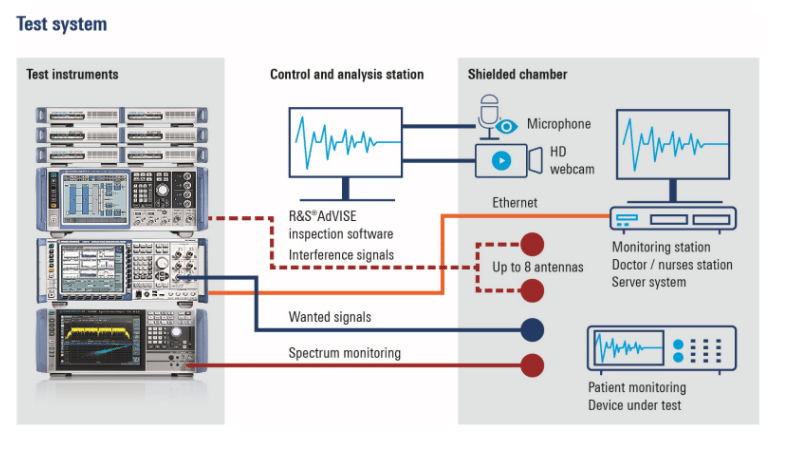
It needs to be mentioned that additional power amplifiers and high gain directional horn antennas are required in order of boost up the signal level from the device under test as required; for the inspection software to work properly, illumination may also be required. The entire test is performed inside a fully anechoic chamber in order to block out all electromagnetic signals from the surrounding environment and make the coexistence measurement fully controlled and re-reproducible.
A fully compliant product gives its users more confidence. In this modern connected world and with more and more connected products joining the ecosystem every day, we should test medical products today using the RF spectral reality of tomorrow, in order to future proof the wireless performance of a device throughout its life time.
Bio
Naseef Mahmud received his Master’s Degree in Electrical, IT and computer engineering with a focus on communication engineering from the RWTH Aachen University in Germany in 2014. Since then he has been working as an application development engineer at the test & measurement company, Rohde & Schwarz. In this role, Naseef had the unique opportunity to work with and gain industry insights from some of the biggest companies in multiple industries. He holds multiple patents in the field of satellite, IoT and automotive testing. Over the years, Naseef has become a trusted advisor for the topic of over the air testing and coexistence testing for Rohde & Schwarz as well as their customers. He also represents Rohde & Schwarz at the REDCA and ANSI C63.27 wireless coexistence work group.