Filters are some of the most common components found in wireless system designs. They are typically used to block interference from various sources. As limited spectrum resources are allocated to ever increasing number of users, more conflicts between these users arise and more filters are constantly needed. Nowadays, interference is quite common between cellular base stations, satellite systems, radar installations, and other type of access and backhaul communications systems. In many cases, traditional filters are unable to cope with the requirements, typically due to insufficient guard band. For example, in some parts of the world, LTE base stations and satellite receivers share the L-Band frequencies. And at around 3.5GHz, 5G operators, CBRS radios, and military radars are trying to co-exist. To address this in-band interference, a new, tunable filtering technology is making its presence known in the market, uniquely blending the best of both analog and digital technologies.
Analog Filters
The wireless industry has benefitted from decades of technology advances—bringing dramatic improvements in products and services for operators, enterprise users, and consumers. This includes the evolution from vacuum tubes to transistors to integrated circuits; the development of trunked, cellular, and digital radio systems; and the rise of unlicensed devices for consumers.
However, analog RF filters, critical for combating interference and ensuring efficient use of radio spectrum resources, are still based on analog-era technology with significant performance, size/weight, and manufacturing limitations.
Conventional radio filters require a transition or guard band—a range of frequencies over which losses decrease from the rejection band (where unwanted signals are blocked) to the pass band (where desired signals are allowed to pass). In practice, a guard band is a stretch of spectrum that must be sacrificed to accommodate the coarse behavior of conventional filters.
Another limitation of conventional analog filters is that they are inherently frequency-specific—they must be designed and manufactured for each specific application. If an application requires rejecting signals on different frequencies at different times, then a filter bank—a device containing multiple filters that can be switched in and out of the circuit—may be required.
The biggest problem with conventional filters, however, is that they often fail to provide enough signal rejection. This can happen for a number of reasons: the interfering signal is too close in frequency to the desired signal, the source of the interfering signal is too close to the receiver, or the interfering signal is simply too powerful.
Digital Filters
Digital filters, for example based on finite impulse response (FIR) or infinite impulse response (IIR) architectures, are widely available and are commonly used as part of practically any digital sub-system. They offer immense flexibility in their ability to shape the signal. By nature, these filters operate on digital samples of the original analog signal. In other words, before any digital manipulations can be applied to the signal, it must be sampled and converted to digital representation. This process is not only time consuming but it also degrades the resolution (the dynamic range) of the original signal since analog-to-digital converters (ADCs) have only finite number of bits of resolution. Once available in digital representation, the digital filter (that is essentially a set of mathematical manipulations of the samples) can be applied. This process, again, takes time since every addition or multiplication consumes CPU cycles. Inherently, digital processing introduces latency in the data path that must be accounted for in the application. This often represents a challenge to RF applications given the propagation speed of the native analog signal. Finally, if the desired output of the system needs to be an RF analog signal (like in any transmitter), the digital samples have to be converted yet again, this time back to analog representation using digital-to-analog Converters (DACs). This further contributes to undesirable latency.
Software-Defined Analog Filters
The ideal filter would combine the no-latency, no-sampling characteristics of analog devices with the configurability and flexibility of digital implementations. The industry has been slowly progressing toward digitally-controlled analog devices. For example, digitally controlled attenuators and phase shifters are widely used in radars and other beam-forming applications. To implement filters, attenuators and phase shifters are not enough – delay elements are also necessary, for instance as part of an IIR or FIR structures.
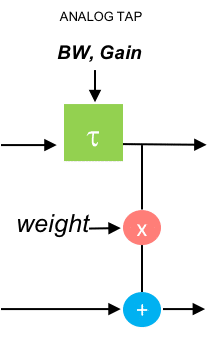
Analog delay elements are difficult to miniaturize – they typically rely on materials where waves are propagating slower than typically used conductors. The choice of material is a delicate balance between insertion loss and physical size/weight. Clearly, such solutions are not possible to implement in a low-cost CMOS IC. This is especially true for wireless applications where propagation delays necessitate long delay elements in the range of hundreds of nanoseconds or even microseconds, in aggregate.
As the only commercially available solution to embed long delay elements in a standard CMOS chip, the Kumu Networks KU1500 RFIC changes the existing paradigms.
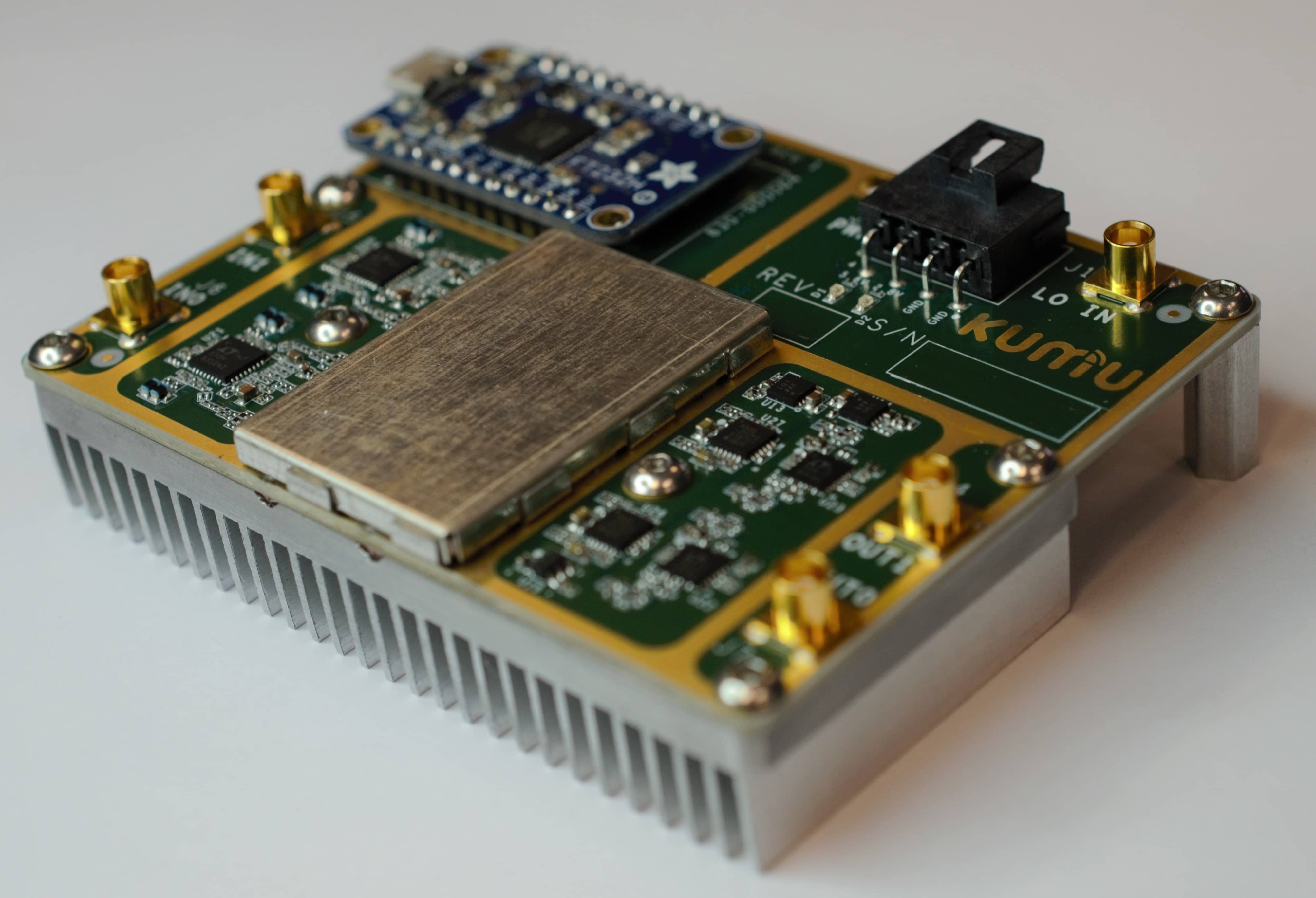
It is truly the first software-controlled analog filter designed for wireless applications. The chip is essentially a multi-tap implementation, in which each tap packs an adder, multiplier, and delay element.
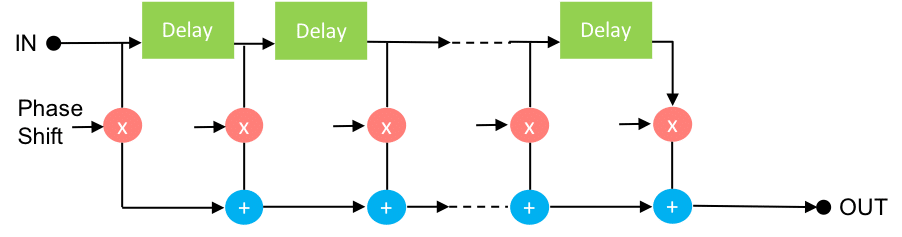
By adjusting the weights of its multipliers through a digitally-controlled API, any desired filter response can be configured.
The software-defined analog filter was originally developed for Self-Interference Cancellation applications where the interferer is co-located next to the receiver. This is achieved by continuously modifying the response of the multi-tap analog filter to match the self-interference, using a tuning logic that estimates the self-interference channel. Traditional filter applications are obviously much simpler than that, and can be handled with static configuration of the filter for the desired response.
Summary
For many applications conventional filters are inexpensive, reasonably small, and do an adequate job. But in other applications, conventional filters fall short.
Digitally-controlled analog filter, like the KU1500, has tremendous potential in environments where guard bands are unavailable or where the cost of the spectrum calls for their elimination. Such filters could contribute dramatically to improved spectrum utilization. Furthermore, self-interference cancellation based on such filters offers the ultimate solution for packing multiple co-located radios into a small form-factor device, while maintaining complete frequency agility across the entire band. Using the technology, radios can communicate simultaneously using nearby, immediately adjacent, or even overlapping frequencies, a problem that actually occurs, for example, in mass-market devices supporting both Wi-Fi and Bluetooth.