An electrical product should be able to ride through any regular voltage sag.
Andreas Eberhard, Power Standards Labs, Alameda, California, USA
Immunity from voltage sags is vital for reliable operation of our ever-more-sophisticated electronic controls and equipment. Every electrical product should be able to ride through typical voltage sags, but in many cases the first sag test occurs after equipment is installed and in operation. Selecting the appropriate sag immunity specification and equipment compliance testing is vital to equipment reliability and customer satisfaction.
Modern equipment can be sensitive to brief disturbances on utility power mains. Electrical systems are subject to a wide variety of power quality problems that can interrupt production processes, can affect sensitive equipment, and may cause downtime, scrap, and capacity losses. The most common disturbance, by far, is a sag—i.e. a brief reduction in voltage lasting a few hundred milliseconds.
Sags are commonly caused by fuse or breaker operation, motor starting, or capacitor switching, but they are also triggered by short circuits on the power distribution system caused by such events as snakes slithering across insulators, trenching machines hitting underground cables, and lightning ionizing the air around high-voltage lines. Many utilities report that 80 percent of electrical disturbances originate within the user’s facility.
A decade ago, the solution to voltage sags was to try to fix them by storing enough energy somehow and then releasing it onto the AC mains when voltage dropped. Some of the old solutions included an uninterruptible power supply (UPS), flywheels and ferroresonant transformers.
More recently, engineers have realized that voltage sag is really a compatibility problem with at least two classes of solutions—viz. you can improve the power, or you can make the equipment tougher. The latter approach is called “voltage sag immunity,” and it’s becoming increasing important around the globe.
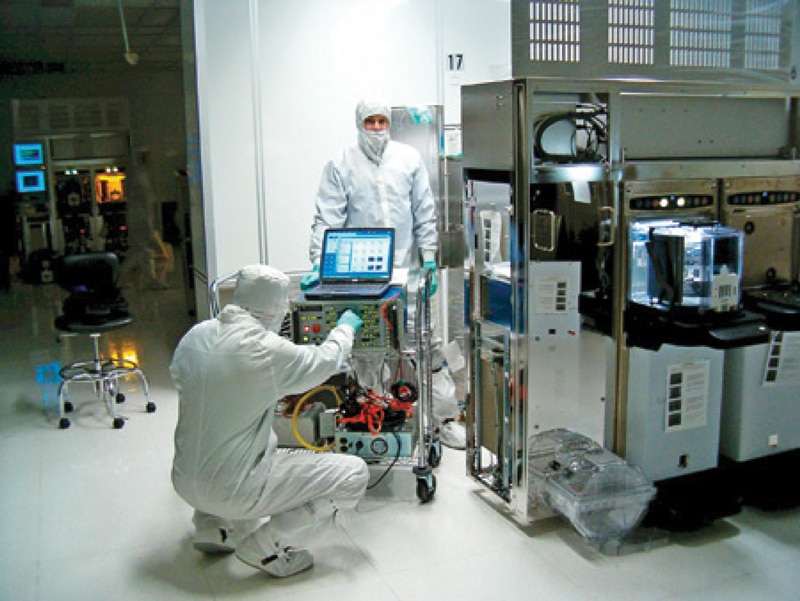
STANDARDS DEVELOPED
Three main primary voltage sag immunity standards are discussed in the following paragraphs: IEC 61000-4-11, IEC 61000-4-34, and SEMI F47. There are others in use—such as IEEE 1100, CBEMA, ITIC, Samsung Power Vaccine, international standards, and MIL-STD; but the first three seem to have the widest acceptance in the marketplace.* IEC 61000-4-11 and IEC 61000-4-34 are a closely related set of standards that cover voltage sag immunity. IEC 61000-4-11 Ed. 2 covers equipment rated at 16 amps per phase or less while IEC 61000-4-34 Ed. 1 covers equipment rated at more than 16 amps per phase. The latter was written after IEC 61000-4-11 so it seems to be more comprehensive.
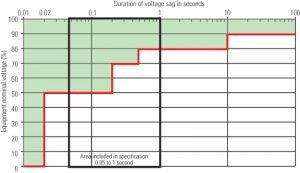
SEMI F47 is the voltage sag immunity standard used in the semiconductor manufacturing industry, where a single voltage sag can result in the multi-million-dollar loss of product if a facility is not properly protected. The semiconductor industry has developed specifications for its manufacturing equipment and for components and subsystems in that equipment. Enforcement is entirely customer-driven in this industry, as semiconductor manufacturers understand the economic consequences of sag-induced failures and generally refuse to purchase new equipment that fails the SEMI F47 immunity requirement. SEMI F47 is currently going through its five-year revision and update cycle. See testers at work in Figure 1.
All three standards specify voltage sags with certain depths and durations for the equipment under test (EUT). For example, a specification may state 70 percent of nominal for 500 milliseconds. The percentage is the amount of voltage remaining, not the amount that is missing. Each standard specifies pass-fail criteria for the EUT when a voltage sag is applied; the IEC standards have a range of pass-fail criteria, but the SEMI F47 standard is more explicit (Figure 2).
A DIFFERENT KIND TESTING
In contrast to most other emissions and immunity testing, voltage sag testing requires the engineer to control and to manipulate all of the power flowing into the EUT. For smaller devices such as personal computers, this requirement is not a great challenge. With larger industrial equipment, perhaps rated at 480 volts three-phase at 200 amps per phase, with an expected inrush current of 600 amps or more, the situation is more complicated. The test engineer must be prepared for common failure mechanisms caused by voltage sags.
The most common failure mechanism is lack of energy. This problem can manifest itself in something as simple as insufficient voltage to keep a critical relay or contactor energized, or as something as complex as an electronic sensor with a failing power supply giving an incorrect reading, which would cause EUT software to react inappropriately.
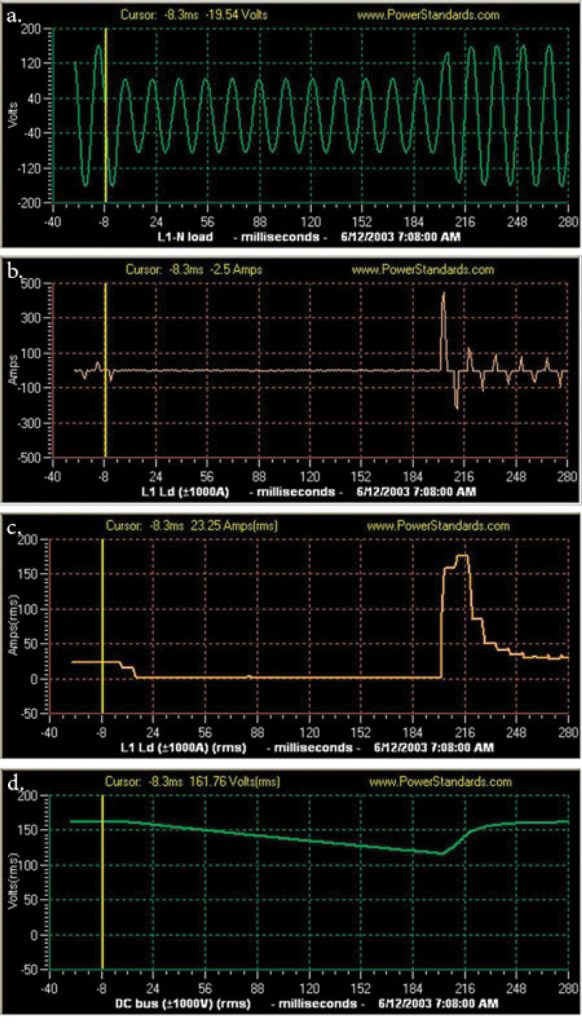
The second most common failure mechanism, surprisingly, occurs just after the sag has finished. In such cases, all of the bulk capacitors inside the EUT recharge at once, causing a large increase in AC mains current. This increase is capable of tripping circuit breakers, opening fuses, and even destroying solid-state rectifiers. Most design engineers correctly protect against this inrush current during power cycling, but many do not consider the similar effects of voltage sags. Care must be taken as the test procedure is developed. A misleading, inaccurate pass for the EUT will result if a sag generator that lacks sufficient current to blow a fuse or to trip a circuit breaker in a half cycle is used. Another common EUT failure mechanism occurs when a sensor detects the voltage sag and proceeds to shut down the EUT. In a straightforward example, a three-phase EUT might have a phase-rotation relay that incorrectly interprets an unbalanced voltage sag as a phase reversal and then shuts down the EUT. A more atypical example would be if you had an airflow sensor mounted near a fan, it detected that the fan had slowed down momentarily, and the equipment software misinterpreted the message from this sensor as indicating that the EUT cooling system had failed. In this case, a software fan failure signal delay is the solution for improving sag immunity.
One more typical EUT failure mechanism involves an uncommon sequence of events. For example, in one case, voltage sag was applied to the EUT, and its main contactor then opened with a bang. Further investigation revealed that a small relay, wired in series with the main contactor coil, actually opened because it received an open relay contact from a stray water sensor. That sensor, in turn, opened because its small 24-VDC supply output dropped to 18 V during the sag. The solution was an inexpensive bulk capacitor across the 24-VDC supply.
Many other failure mechanisms can take place during voltage sags. The question for the test engineer remains, “How do we fix this problem?” Usually, there is a simple, low-cost fix once the problem is identified. (Note the various modes of failure mechanisms depicted in Figure 3.)
There is a new way to increase the reliability of products despite common power quality and voltage sag problems. Imagine a more “intelligent” product that would vary its performance depending on the severity of the voltage sag problem. Expect to see more and more of such permanently installed power quality monitors in use at the product level. This new embedded technology comes with the huge advantage of allowing the evaluation power quality problems and energy consumption directly within the product or machine. A new power quality monitor as shown in Figure 4 has the same functionality and features as more well-known expensive power quality instruments. The latter are typically used on a facility level and are clearly too expensive and far too big to integrate and design into products.

Traditional power quality instruments have been designed with their own unique packaging. In today’s electronics industry, however, the trend is toward embedded and intelligent functionality. This holds true for power quality measurement as well. By packaging this new smaller power quality instrument in a standard 35mm DIN-rail circuit breaker package, installation is greatly simplified. The newer smaller devices will detect any kind of power problems on the AC lines and will interact directly with the product, machine, or facility personnel.
Some versions have Ethernet connectivity— including a web server, an FTP server, and an e-mail generator—wireless radio connectivity, and a modem connection. Conversely, these devices can be optimized to function without connectivity. A device can easily store a year’s data on a removable SD memory card. In devices modelled on the digital camera design, the power quality device and monitor will require absolutely no software. Recall that when a digital camera is connected to a computer, the pictures appear immediately in a folder on the disk drive. This same “instant-on” feature holds true for these small power quality measurement devices.
CONCLUSION
With the ever-increasing use of sophisticated controls and equipment in industrial, commercial, institutional, and governmental facilities, the continuity, reliability, and quality of electrical service has become extremely crucial to many power users. Power quality is unlikely to improve in the future so the ultimate goal for any product manufacturer is to make its product immune to voltage sags. Just as all modern cars should be able to drive over regular bumps on the road, every electrical product should be able to ride-through any regular voltage sags that will occur despite of the best power that a facility can provide.
END NOTES
*IEC is the International Electrotechnical Commission; SEMI is the Semiconductor Equipment and Materials Institute; CBEMA is the Computer Business Equipment Manufacturers Association; ITIC is the Information Technology Institute Council; and MIL-STD denotes a U.S. Defense Department’s specification.)
Andreas Eberhard is member of various power quality standard committees around the world. Over 10 years of experience in product compliance based on international standards. He is Vice President of Technical Services at Power Standard Labs and can be contacted at [email protected],or +1-510-522-4400.